the Creative Commons Attribution 4.0 License.
the Creative Commons Attribution 4.0 License.
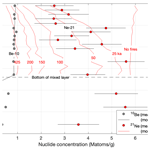
Short communication: Cosmogenic noble gas depletion in soils by wildfire heating
Greg Balco
Alan J. Hidy
William T. Struble
Joshua J. Roering
Measurements of cosmic-ray-produced beryllium-10, neon-21, and helium-3 in quartz in a soil profile from a forested landscape in the Oregon Coast Range show that the cosmogenic noble gases 21Ne and 3He are depleted relative to 10Be in the shallow subsurface. The noble gases are mobile in mineral grains via thermally activated diffusion and 10Be is not, implying that noble gas depletion is the result of surface heating by wildfires and subsequent mixing of partially degassed quartz downward into the soil. Cosmogenic noble gas depletion by wildfire heating of soils is a potential means of estimating wildfire intensity and/or frequency over pre-observational timescales.
- Article
(2201 KB) - Full-text XML
-
Supplement
(486 KB) - BibTeX
- EndNote
Interactions of high-energy cosmic rays with surface rocks and minerals generate a variety of trace nuclides that are not produced by any other natural process. Because the cosmic-ray flux is rapidly attenuated with depth below the Earth's surface, these cosmic-ray-produced nuclides are a signature of surface exposure. Measurements of their concentrations are commonly used to measure the times, rates, and durations of geologic processes that act to form and degrade the Earth's surface, most commonly by “surface exposure dating” of surfaces or landforms created by processes such as glacier and ice sheet advance and retreat, river erosion, or fault movement (Dunai, 2010).
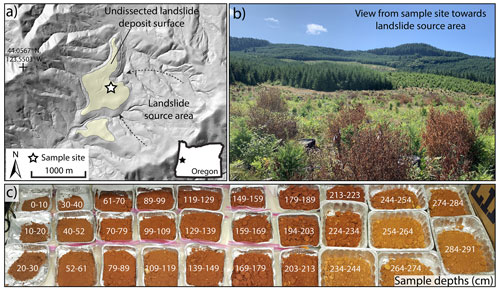
Figure 1Cougar Pass sample site. (a) Lidar hillshade of Cougar Pass landslide, showing the landslide deposit and source area and the sample location within the landslide deposit. (b) View of the landslide source area looking southeast from sample site. (c) Samples from soil profile, labeled with depth in centimeters. Not all of the samples shown in the photo were used for cosmogenic-nuclide measurements; see Table S1 in the Supplement for details.
Commonly measured cosmic-ray-produced nuclides include both radionuclides (e.g., beryllium-10, aluminum-26) and stable noble gases (neon-21, helium-3). Although these are produced by similar nuclear reactions, their behavior after production is very different. 10Be and 26Al produced from Si and O in quartz (SiO2) are tightly bound in the mineral lattice and can only be extracted by complete dissolution of the mineral. 21Ne and 3He, on the other hand, are produced by the same processes in the same mineral but are mobile within the mineral lattice and can be lost by thermally activated diffusion (Brook and Kurz, 1993; Shuster and Farley, 2005; Tremblay et al., 2014). Because of this behavior, noble gases (He, Ne, Ar) produced not from cosmic rays but from decay of naturally occurring U, Th, and K in many common minerals are often used to reconstruct the thermal history of rocks and minerals in the subsurface (Reiners et al., 2005). Likewise, the comparison of immobile and diffusively mobile cosmic-ray-produced nuclides can reveal aspects of the thermal history that samples have experienced during surface and near-surface exposure. This idea has been applied for paleoclimate reconstruction (Tremblay et al., 2014, 2019; Gribenski et al., 2022), and in this paper we show another potential application in estimating wildfire frequency and/or intensity over timescales longer than the observational record. Although it is already well known that rock surface heating by natural or human-caused fire results in loss of radiogenic He and Ar (Gillespie et al., 1989; Mitchell and Reiners, 2003; Reiners et al., 2007), this has most commonly been viewed as a “bug” that is a potential source of error in noble gas geochronology and thermochronology. We show that applying the same idea to cosmogenic noble gases is potentially a “feature” that could be used for understanding long-term wildfire regimes.
We collected cosmogenic 10Be, 21Ne, and 3He data from a site at Cougar Pass (44.053∘ N, 123.531∘ W, 250 m elevation) in the Oregon Coast Range (Fig. 1). The heavily forested, soil-mantled landscape of the Oregon Coast Range is underlain by the Tyee Formation, a relatively undeformed Eocene turbidite sandstone (Heller and Dickinson, 1985). Important landscape-forming processes in the region include (i) bioturbation via tree rooting and turnover of the closed-canopy coniferous forest, which roughens the land surface and contributes to the conversion of bedrock to soil (Roering et al., 2010), as well as (ii) extensive mass wasting by shallow and deep-seated landslides, which generate low-relief, bench-like terrain (Roering et al., 2005). The Cougar Pass site is located atop an ancient landslide deposit identified by Baldwin and Howell (1949) as a point of drainage capture that now separates streams draining to the Pacific Ocean and the Willamette Valley. Our soil profile is in the interior of the landslide deposit, which has a broad, flat surface that displays a deep, highly weathered soil. Soil development, as well as comparisons to roughness-dated landslides in the region, indicate that the site has been stable with minimal surface erosion for >200 ka (LaHusen et al., 2020). Paleoenvironmental archives indicate that regional forest composition has varied significantly on glacial–interglacial timescales between an subalpine, open-parkland-dominated forest during glacial periods and a closed-canopy Douglas Fir forest, as is present now, during interglacials (Worona and Whitlock, 1995). Wildfire has been common in the region throughout shifts in forest composition, although it has been modulated by regional climate variations associated with sea-surface temperatures and seasonal moisture availability via winter storm tracks and summer subtropical high pressure (Long et al., 2018).
We obtained soil samples at 10 cm depth increments by auger sampling at the Cougar Pass site, and measured concentrations of cosmogenic 10Be, 21Ne, and 3He in 0.25–0.5 mm diameter quartz grains extracted from these samples (see the Supplement). All three of these nuclides have similar production systematics, being dominantly produced by fast neutron spallation on Si and O (10Be and 3He) or Si only (21Ne). Thus, although their absolute production rates in quartz differ (the production ratio of 21Ne to 10Be is 4.05 and that of 3He to 21Ne is approximately 7), the exponential decrease in the production rate with depth characteristic of spallogenic production is the same. However, their behavior during heating is very different. 10Be is not diffusively mobile and is retained during heating. 21Ne is not mobile at ambient surface temperatures but is lost from quartz grains over geologic timescales at temperatures above approximately 90 ∘C and over timescales of minutes to hours at 300–400 ∘C. 3He is more diffusively mobile than 21Ne. Cosmogenic 3He is continually lost by diffusion even at ambient Earth surface temperatures and lost within minutes to hours at temperatures above ∼150–200 ∘C (Shuster and Farley, 2005; Tremblay et al., 2014).
10Be concentrations in the soil profile (Fig. 2) show a well-mixed layer with nearly constant 10Be concentration between the surface and 170 cm depth. Surface layers with well-mixed cosmogenic-nuclide concentrations are characteristic of vegetated landscapes subject to vertical soil mixing by rooting, tree throw, and similar processes (e.g., Perg et al., 2001; Rovey and Balco, 2015). This example is unusually deep and unusually homogeneous, although deep mixing is expected from studies of root depth in the Oregon Coast Range (e.g., Roering et al., 2010). An approximate estimate of the exposure age of the surface derived from the mean 10Be concentration (8.5 × 105 atoms g−1) and mean production rate (2.2 atoms g−1 yr−1) in the mixed layer is 425 ka, although this is likely not a precise age estimate because it assumes zero surface erosion (which could result in an underestimate of the age) and zero inherited 10Be (which could result in an overestimate). As 21Ne and 3He have the same production systematics as 10Be, we would expect 21Ne and 3He concentrations to show a similar mixed-layer profile. However, we do not observe this. Instead, 21Ne and 3He concentrations are lowest at the surface and increase with depth through the mixed layer.
Because we have measured 10Be, 3He, and 21Ne in the same quartz samples and these nuclides have the same production systematics, the differences in their depth profiles that we observe are impossible in a geochemically closed system. Thus, quartz in the soil profile is not a closed system. The facts that (i) both 21Ne and 3He are increasingly depleted relative to 10Be with proximity to the surface and (ii) 3He, which is more diffusively mobile than 21Ne, is more depleted than 21Ne, imply that open-system behavior is due to 21Ne and 3He loss by surface heating. As the site has a known history of wildfire, it is nearly certain that 21Ne and 3He depletion is the result of wildfire heating.
The depth of 21Ne and 3He depletion far exceeds the depth of expected wildfire heating to temperatures needed to degas Ne from quartz. Wildfire heating calculations (e.g., Richter et al., 2022) typically predict temperatures up to 600 ∘C at the soil surface, decreasing approximately exponentially with depth with an attenuation length of 5–10 cm. Significant 21Ne loss is only expected to occur within the uppermost ∼10 cm of the soil. Therefore, 21Ne and 3He depletion to more than 2 m depth requires that quartz is being degassed at the surface and then mixed down into the soil profile. This is not surprising, because well-mixed 10Be concentrations show that vertical mixing is fast relative to nuclide production. However, it means that 21Ne and 3He concentrations in the soil retain a record of more than just the most recent heating event. This is an important contrast with observations of noble gas depletion by fire in rock surfaces (Gillespie et al., 1989; Mitchell and Reiners, 2003) because in the absence of subsurface mixing, noble gas depletion is confined to a thin surface layer, and a single heating event could destroy any evidence of previous heating events. Deep vertical mixing allows the soil to retain a memory of many heating events rather than just one.
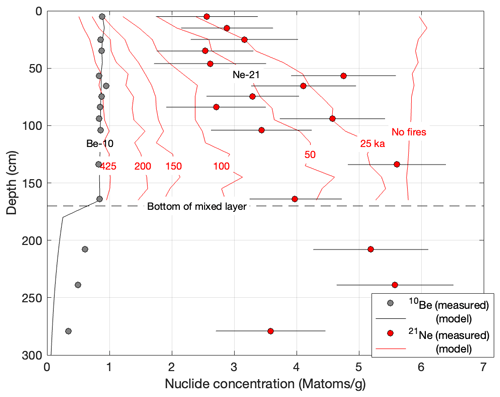
Figure 3Simplified model simulation for 10Be and 21Ne accumulation and loss in a mixed soil. Exposure time and mixing rate are chosen to match measured 10Be concentrations in the mixed layer (although not below the mixed layer; see Sect. 4). Model 21Ne concentrations in the mixed layer are calculated for the same exposure time and mixing rate, but different onset times (given for each line in ka) for the simulated fire regime. For example, the line marked “50 ka” is the result of a simulation in which the fire regime starts 50 000 years before present.
Finally, the observation that noble-gas-depleted quartz is not fully mixed to the bottom of the mixed layer identified from 10Be concentrations requires that the system is not in steady state. If both mixing and heating had been steady and continuous since the formation of the soil, all nuclides would be similarly homogenized within the mixed layer. They are not, so heating intensity must have increased at some time after soil formation. We highlight this effect with a simplified one-dimensional model of 10Be and 21Ne accumulation and loss in a mixed soil (we omit 3He because 3He is continuously diffusively lost at ambient temperature even without fire heating, so a more complex model with additional paleoclimate assumptions is required).
The model simulation, which is described in the Supplement and the results of which are shown in Fig. 3, considers a large number of soil particles that describe random walks vertically through a 170 cm mixed layer and computes 10Be production as a function of depth for each step in the random walk. A model duration of 425 ka (see above) and random walk distance of 0±10 cm in each 250-year time step replicates 10Be concentrations in the mixed layer. The model underestimates 10Be concentrations below the mixed layer, which indicates that the assumption that vertical mixing stops abruptly at 170 cm is oversimplified, but this does not affect the behavior within the mixed layer that the model is intended to simulate. We similarly compute 21Ne production in the same particles but also assume that during each time step there is one wildfire with a 1 h duration, a surface temperature of 450 ∘C, and an exponential attenuation depth of 6 cm (this is a characteristic scenario from Richter et al., 2022). Each quartz grain loses 21Ne as a single spherical diffusion domain (Fechtig and Kalbitzer, 1966) with temperature-dependent diffusivity as measured by Shuster and Farley (2005). It is also necessary to assume a background concentration of 21Ne, which has not been measured for the soil protolith, and thus is chosen to yield an approximate fit to the data. This scenario is roughly based on late Pleistocene fire frequency as reconstructed by Long et al. (2018), but it is not unique: the same diffusive loss can be achieved by many combinations of fire frequency, fire duration, and/or temperature profile.
To highlight the effect of transient changes in fire regime, we simulate a change in fire intensity by imposing the fire regime partway through the simulation. As shown in Fig. 3, if there are no fires ever, 21Ne concentrations are high and well mixed. If wildfire heating occurs throughout the entire exposure history of the soil, concentrations are low and well mixed. The observed gradient in 21Ne concentration requires a transient scenario in which the fire regime has recently intensified for a time that is not long enough to permit complete mixing of quartz degassed at the surface down into the soil. This is broadly consistent with the hypothesis developed from paleoenvironmental data that during glacial periods, a parkland meadow-dominated forest generated low-intensity fires, but during interglacial periods a closed-canopy Douglas fir forest generates similarly frequent but higher-intensity fires (Long et al., 2018). Thus, although diffusive processes are inherently information-destroying and therefore transient scenarios that explain the data are unlikely to be unique, a simple model–data comparison shows that cosmogenic noble gas depletion within a deep soil profile can contain information about past changes in fire regime.
Observations of cosmogenic noble gas depletion in soils may have several useful applications in studying wildfire. First, prehistoric wildfire frequency and intensity reconstructions are typically based on charcoal counts in sedimentary deposits (Marlon et al., 2012). This provides an effective reconstruction of fire frequency, but reconstructing fire intensity is indirect and more difficult. Cosmogenic noble gas depletion is complementary to this approach in that, in general, it is unlikely to record individual events but records a combination of aggregate frequency and intensity. If frequency is known from other data, in principle intensity could be inferred. It is also complementary to lake-sediment-based records in that it provides site-specific rather than areally averaged information.
Second, an empirical measure of the long-term average temperature and depth history that has actually been experienced in past wildfires may be useful for buried infrastructure design and protection. Although vertical mixing may complicate this in forested landscapes, many environments (e.g., grasslands) do not experience deep vertical mixing.
Third, cosmogenic noble gas depletion is a potential means of evaluating whether recent wildfires are more intense than fires that took place in a pre-management regime. The depth profile of cosmogenic noble gases should reflect a mean fire intensity integrated over many past fires. If a fire is significantly more intense than past fires, 3He and 21Ne may be systematically more depleted in the shallow subsurface in areas affected by the recent fire than in adjacent areas that were not affected but share the same long-term fire regime.
This approach also has some application challenges. First, there are several technical challenges, including the difficulty of measuring cosmogenic 21Ne at low concentrations in the presence of commonly large inherited or background inventories, as well as the fact that He and Ne diffusion kinetics are variable in quartz from different sources (Tremblay et al., 2014) and may require lithology-specific characterization. Second, as noted above, the fact that diffusion and mixing are inherently information-destroying processes means that it is unlikely that a unique history of millennial-scale changes in fire regime could be reconstructed from a 21Ne or 3He depth profile. On the other hand, this does not affect its potential utility in hypothesis testing: in many cases these data could be clearly consistent or inconsistent with hypotheses about changes in fire regime developed from other paleoenvironmental information.
All measurements described in the paper, as well as MATLAB code implementing the simplified model simulation, are included in the Supplement.
The supplement related to this article is available online at: https://doi.org/10.5194/gchron-6-71-2024-supplement.
WTS and JTR were primarily responsible for fieldwork and sample collection and preparation. AJH and GB were primarily responsible for cosmogenic-nuclide measurements. GB prepared the manuscript with contributions from all authors.
At least one of the (co-)authors is a member of the editorial board of Geochronology. The peer-review process was guided by an independent editor, and the authors also have no other competing interests to declare.
Publisher’s note: Copernicus Publications remains neutral with regard to jurisdictional claims made in the text, published maps, institutional affiliations, or any other geographical representation in this paper. While Copernicus Publications makes every effort to include appropriate place names, the final responsibility lies with the authors.
The authors thank Jerod Aguilar, Annette Patton, and Alianora Walker for help with sample collection and preparation and Dave Cramsey and Roseburg Forest Products for facilitating site access.
This research was supported in part by the Anne and Gordon Getty Foundation. The LLNL contribution to this work was performed under the auspices of the U.S. Department of Energy under contract DE-AC52-07NA23744. This is contribution LLNL-JRNL-852163.
This paper was edited by Yeong Bae Seong and reviewed by Ken Ferrier and M. Akif Sarikaya.
Baldwin, E. M. and Howell, P. W.: The Long Tom, a former tributary of the Siuslaw River, Northwest Sci., 23, 112–124, 1949. a
Brook, E. J. and Kurz, M. D.: Surface-exposure chronology using in situ cosmogenic 3He in Antarctic quartz sandstone boulders, Quat. Res., 39, 1–10, 1993. a
Dunai, T. J.: Cosmogenic nuclides: principles, concepts and applications in the Earth surface sciences, Cambridge University Press, ISBN 1108445721, 2010. a
Fechtig, H. and Kalbitzer, S.: The diffusion of argon in potassium-bearing solids, in: Potassium argon dating, edited by: Schaeffer, O. and Zähringer, J., Springer, 68–107, ISBN 111457709X, 1966. a
Gillespie, A. R., Budinger Jr., F. E., and Abbott, E. A.: Verification of prehistoric campfires by 40Ar 39Ar analysis of fire-baked stones, J. Archaeol. Sci., 16, 271–291, 1989. a, b
Gribenski, N., Tremblay, M. M., Valla, P. G., Balco, G., Guralnik, B., and Shuster, D. L.: Cosmogenic 3He paleothermometry on post-LGM glacial bedrock within the central European Alps, Geochronology, 4, 641663, https://doi.org/10.5194/gchron-4-641-2022, 2022. a
Heller, P. and Dickinson, W.: Submarine ramp facies model for delta-fed, sand-rich turbidite systems, AAPG Bull., 69, 960–976, 1985. a
LaHusen, S. R., Duvall, A. R., Booth, A. M., Grant, A., Mishkin, B. A., Montgomery, D. R., Struble, W., Roering, J. J., and Wartman, J.: Rainfall triggers more deep-seated landslides than Cascadia earthquakes in the Oregon Coast Range, USA, Sci. Adv., 6, eaba6790, https://doi.org/10.1126/sciadv.aba6790, 2020. a
Long, C. J., Power, M. J., and Grigg, L. D.: A 35,000 yr fire history from the Oregon Coast Range, USA, in: From Saline to Freshwater: The Diversity of Western Lakes in Space and Time: Geological Society of America Special Paper 536, edited by: Starratt, S. and Rosen, M., Geol. Soc. Am., ISBN 0813725364, 2018. a, b, c
Marlon, J. R., Bartlein, P. J., Gavin, D. G., Long, C. J., Anderson, R. S., Briles, C. E., Brown, K. J., Colombaroli, D., Hallett, D. J., Power, M. J., Scharf, E. A. and Walsh, M. K.: Long-term perspective on wildfires in the western USA, P. Natl. Acad. Sci. USA, 109, 535–543, https://doi.org/10.1073/pnas.1112839109, 2012. a
Mitchell, S. G. and Reiners, P. W.: Influence of wildfires on apatite and zircon (U-Th)/He ages, Geology, 31, 1025–1028, 2003. a, b
Perg, L., Anderson, R., and Finkel, R.: Use of a new 10Be and 26Al inventory method to date marine terraces, Santa Cruz, California, USA, Geology, 29, 879–882, 2001. a
Reiners, P., Thomson, S., McPhillips, D., Donelick, R., and Roering, J.: Wildfire thermochronology and the fate and transport of apatite in hillslope and fluvial environments, J. Geophys. Res.-Earth, 112, F04001, https://doi.org/10.1029/2007JF000759, 2007. a
Reiners, P. W., Ehlers, T. A., and Zeitler, P. K.: Past, present, and future of thermochronology, Rev. Mineral. Geochem., 58, 1–18, 2005. a
Richter, E. G., Fischer, E. C., Metz, A., and Wham, B. P.: Simulation of heat transfer through soil for the investigation of wildfire impacts on buried pipelines, Fire Technol., 58, 1889–1915, 2022. a, b
Roering, J. J., Kirchner, J. W., and Dietrich, W. E.: Characterizing structural and lithologic controls on deep-seated landsliding: Implications for topographic relief and landscape evolution in the Oregon Coast Range, USA, Geol. Soc. Am. Bull., 117, 654–668, 2005. a
Roering, J. J., Marshall, J., Booth, A. M., Mort, M., and Jin, Q.: Evidence for biotic controls on topography and soil production, Earth Planet. Sc. Lett., 298, 183–190, 2010. a, b
Rovey, C. W. and Balco, G.: Paleoclimatic interpretations of buried paleosols within the pre-Illinoian till sequence in northern Missouri, USA, Palaeogeogr. Palaeocl., 417, 44–56, 2015. a
Shuster, D. L. and Farley, K. A.: Diffusion kinetics of proton-induced 21Ne, 3He, and 4He in quartz, Geochim. Cosmochim. Ac., 69, 2349–2359, 2005. a, b, c
Tremblay, M. M., Shuster, D. L., and Balco, G.: Diffusion kinetics of 3He and 21Ne in quartz and implications for cosmogenic noble gas paleothermometry, Geochim. Cosmochim. Ac., 142, 186–204, 2014. a, b, c, d
Tremblay, M. M., Shuster, D. L., Spagnolo, M., Renssen, H., and Ribolini, A.: Temperatures recorded by cosmogenic noble gases since the last glacial maximum in the Maritime Alps, Quat. Res., 91, 829–847, 2019. a
Worona, M. A. and Whitlock, C.: Late Quaternary vegetation and climate history near Little Lake, central Coast Range, Oregon, Geol. Soc. Am. Bull., 107, 867–876, 1995. a
- Abstract
- Cosmogenic radionuclides and noble gases
- Soil profile at Cougar Pass, Oregon, USA
- Cosmogenic-nuclide depth profiles at Cougar Pass
- Near-surface depletion of cosmogenic noble gases
- Useful applications
- Code and data availability
- Author contributions
- Competing interests
- Disclaimer
- Acknowledgements
- Financial support
- Review statement
- References
- Supplement
- Abstract
- Cosmogenic radionuclides and noble gases
- Soil profile at Cougar Pass, Oregon, USA
- Cosmogenic-nuclide depth profiles at Cougar Pass
- Near-surface depletion of cosmogenic noble gases
- Useful applications
- Code and data availability
- Author contributions
- Competing interests
- Disclaimer
- Acknowledgements
- Financial support
- Review statement
- References
- Supplement