the Creative Commons Attribution 4.0 License.
the Creative Commons Attribution 4.0 License.
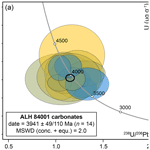
In situ U–Pb dating of 4 billion-year-old carbonates in the martian meteorite Allan Hills 84001
Romain Tartèse
Ian C. Lyon
In situ carbonate U–Pb dating studies have proliferated dramatically in recent years. Almost all these studies have targeted relatively young terrestrial calcite up to Carboniferous in age. To assess the robustness of the carbonate U–Pb chronometer in deep time, we carried out in situ U–Pb analyses in magnesite–ankerite–calcite carbonates in the martian meteorite Allan Hills (ALH) 84001. Carbonates in ALH 84001 formed at ca. 3.94 Ga, and there is little evidence that much happened to this rock since then, making it an ideal sample to test the robustness of the U–Pb system in old carbonates. We obtained a concordant date of 3941 ± 49/110 Ma (n=14, MSWD = 2.0), which is identical to the step-leaching date determined previously. These results thus confirm that old carbonates are amenable to U–Pb dating in samples that have had a relatively simple history post-carbonate formation.
- Article
(1720 KB) - Full-text XML
-
Supplement
(1667 KB) - BibTeX
- EndNote
Analytical developments in laser ablation inductively coupled plasma mass spectrometry (LA-ICP-MS) over the last decade have driven important progress in in situ dating of carbonates – particularly of calcite and, occasionally, dolomite – using the radioactive decay of uranium (U) into lead (Pb) (see the recent review by Roberts et al., 2020, for example). Indeed, biogenic, diagenetic, and vein carbonates can typically incorporate up to ca. 10–20 µg g−1 U and up to ca. 100 µg g−1 U in speleothems (e.g. Roberts et al., 2020). Carbonates typically also incorporate initial Pb, meaning that multiple analyses on carbonate samples often yield linear arrays in a Tera–Wasserburg inverse concordia diagram, providing information on both the composition of the initial Pb and the age of formation of the carbonates. Recent applications of carbonate U–Pb dating using LA-ICP-MS include constraining the timing of sedimentation, lithification, and diagenesis (e.g. Drost et al., 2018; Godeau et al., 2018; Mueller et al., 2020; Brigaud et al., 2021); faulting (e.g. Ring and Gerdes, 2016; Roberts and Walker, 2016; Goodfellow et al., 2017; Nuriel et al., 2017, 2019; Hansman et al., 2018; Beaudoin et al., 2018; Holdsworth et al., 2019; Smeraglia et al., 2019); aragonite-to-calcite conversion in ammonites (Li et al., 2014); alteration of oceanic crust (Coogan et al., 2016); veining, hydrothermalism, and mineralisation (Burisch et al., 2017, 2018; Parrish et al., 2018; Walter et al., 2018; Bertok et al., 2019; Drake et al., 2019, 2020; MacDonald et al., 2019); palaeoclimate reconstructions (Nicholson et al., 2020); and hominin dispersion (Scardia et al., 2019), for example.
All these in situ studies have targeted relatively young samples (younger than ca. 465 Ma), with two thirds of the dates being younger than 50 Ma and all but three being younger than 300 Ma (Table S1 in the Supplement). This is consistent with the accepted idea that carbonates are not very resistant to resetting of their U–Pb isotope systematics when thermal- and/or fluid-related alteration events take place after their formation (e.g. Roberts et al., 2020). However, a few studies have focused on dating older carbonate samples, using wet chemistry to isolate Pb isotopes, and obtained isochron dates ranging between ca. 1.60 and 2.84 Ga (Moorbath et al., 1987; Bau et al., 1999; Ray et al., 2003; Sarangi et al., 2004; Fairey et al., 2013). These dates have been interpreted as dating the deposition of these carbonates, suggesting that, in some settings, the Pb isotope systematics of carbonates can remain undisturbed for billions of years. To further assess the robustness of the carbonate U–Pb chronometer in deep time, we decided to attempt in situ LA-ICP-MS U–Pb dating of carbonates in the martian meteorite Allan Hills 84001 (ALH 84001). The formation of these carbonates has been dated at 3.94 ± 0.02 Ga (2σ) using Rb-Sr analyses on acid leachates via thermal ionisation mass spectrometry (Borg et al., 1999; Beard et al., 2013; date recalculated using a 87Rb decay constant of 1.3972 × 10−11 a−1; Villa et al., 2015).
The meteorite ALH 84001 is an orthopyroxenite – a cumulate rock mostly comprising orthopyroxene, olivine, and chromite (e.g. Mittlefehldt, 1994) – which formed 4.09 ± 0.03 Ga ago, as suggested by Lu-Hf and dating (Bouvier et al., 2009; Lapen et al., 2010). Carbonate-rich areas are irregularly scattered throughout ALH 84001, appearing as spherical or hemispherical globules, discs along fractures, and irregular fillings in orthopyroxene (see review by Treiman, 2021, and references therein). The patches of carbonates show strong compositional zoning, ranging from calcite-rich to magnesite–siderite solid solution compositions (e.g. Corrigan and Harvey, 2004; Holland et al., 2005). These carbonates likely formed at low temperature (ca. 10–20 ∘C; Halevy et al., 2011; del Real et al., 2016) during fluid–rock interactions that were broadly contemporaneous with the main shock event recorded in ALH 84001 (e.g. Treiman, 2021). In order to test the robustness of the U–Pb system in ca. 4 Ga-old carbonates, it is essential to understand the geological history of those carbonates to assess whether they have been affected by any hydrous or other alteration event since their formation.
Dating carbonate-rich fractions in ALH 84001 using the Rb-Sr system yielded a precise formation age of 3.94 ± 0.02 Ga (2σ; Borg et al., 1999; Beard et al., 2013), which is consistent with a less precise isochron corresponding to a date of 4.045 ± 0.090 Ga (2σ; Borg et al., 1999). The formation of these carbonates occurred at a low temperature (< 20 ∘C) and likely involved the mixing of two different water sources, one rich in Ca, the other rich in Fe (e.g. Halevy et al., 2011, del Real et al., 2016; Bridges et al., 2019, Treiman, 2021). These carbonate-forming fluids equilibrated with the atmosphere at the time, unlike igneous minerals in the matrix (Shaheen et al., 2015). A contemporaneous impact event raised the temperature of the surrounding plagioclase to ca. 1400 ∘C, melting it to produce glass and faulting carbonate globules (Mittlefehldt, 1994). Following this, Treiman (2021) states “there is little evidence that anything had happened to ALH 84001 since 3.9 Ga” – until another impact event at ca. 14 Ma, which caused the progenitor material that formed ALH 84001 to be ejected from Mars (Eugster et al., 1997). After ca. 14 Ma in space, ALH 84001's parent meteoroid fell to Antarctica ca. 13 000 years ago (Eugster et al., 1997) and remained buried deep in the ice for millennia, only emerging at the surface of the Allan Hills ice field probably no more than 500 years ago (Krähenbühl et al., 1998). Finally, there is extensive olivine and glass of plagioclase composition in ALH 84001, with no evidence of any alteration to clays or phyllosilicates. Modelling of Ar diffusion within the constituent minerals implies that the progenitor material for ALH 84001 was not subjected to temperatures > 30 ∘C for any “long duration” (Cassata et al., 2010; Shuster and Weiss, 2005). All these lines of evidence indicate that the minerals in ALH 84001 were not exposed to hydrous fluids or temperatures > 25–30 ∘C during the last 3.9 Ga (e.g. Treiman, 2021).
The studied polished section (Fig. 1) was derived from a chip of the ALH 84001,287 allocation from the NASA Ancient Mars Meteorite Program. The section contains patches of carbonates, associated with chromite, in between larger orthopyroxene grains (Fig. 1). The carbonates display the range of compositions typical for ALH 84001 carbonates, from Mg-rich magnesite to Ca-rich calcite, with intermediate Fe-rich ankerite areas (Fig. 1). The Mg- and Fe-rich carbonates seem to be part of broken rosettes, while the Ca-rich carbonates appear to be associated with maskelynite (Fig. 1).
U–Pb analyses were carried out at the University of Manchester using a Teledyne Photon Machines Analyte Excite+ 193 nm ArF excimer laser ablation system equipped with a HelEx II active two-volume ablation cell coupled to an Agilent 8900 triple-quadrupole inductively coupled plasma mass spectrometer (ICP-MS) using a signal-smoothing device (see Table S2 for a summary of the analytical setup and data processing procedure).
The material ablated from target carbonates was carried to the ICP-MS by high purity He, which was mixed with Ar before injection into the plasma source. High purity N2 was added to the He stream at a flow rate of 2 mL min−1 to enhance sensitivity. Tuning of the ICP-MS and mass calibration were performed at the start of the analytical session by optimising the ion signals during ablation of the NIST SRM 612 reference glass while maintaining close to unity and minimising the ratio (ca. 0.3 %). Glass and carbonates were ablated using a 25 μm laser beam size, a fluence of 4 J cm−2, and a repetition rate of 5 Hz. Each analysis lasted 50 s and was preceded by 30 s counting time of the gas blank (background). The masses analysed and the corresponding dwell times are reported in Table S2.
The reference glass NIST614 (0.823 µg g−1 U, 2.32 µg g−1 Pb; Jochum et al., 2011) was used to correct for fractionation, while mass bias correction of the measured ratios was carried out using repeated analyses of the reference calcite WC-1, which has a thermal ionisation mass spectrometry (TIMS) age of 254.4 ± 6.4 Ma (Roberts et al., 2017). To ensure accuracy, the Duff Brown Tank (DBT) calcite (64.0 ± 0.7 Ma; Hill et al., 2016) and AUG-B6 calcite (43.0 ± 1.0 Ma; Pagel et al., 2018) were also analysed and used as secondary reference materials. Since there is no magnesite–ankerite carbonate reference material, we have to rely on using a calcite reference material to correct for fractionation in ALH 84001 carbonates.
Data processing was carried out using Iolite v4.5, using the NIST614 glass as a primary reference material to remove instrument baseline contributions, the mass bias of Pb isotopes, and the downhole fractionation and instrumental drift of ratios (Paton et al., 2011). The reproducibility obtained on NIST614 for (± 1.7 %, n=9, 95 % confidence level) and (± 1.6 %, n=9, 95 % confidence level) ratios were propagated by quadrature addition into and individual uncertainties for each analysis. Repeated analyses of the NIST612 glass yielded an average ratio of 0.871 ± 0.044 (n=8, 2 standard deviation), which is within error of its known ratio of 0.90745 ± 0.00004 (Baker et al., 2004).
The data obtained for the reference calcite WC-1 were then plotted in a Tera–Wasserburg diagram using IsoplotR (Vermeesch, 2018) and yielded a lower intercept uncorrected date of 255.2 ± 5.9 Ma (95 % confidence level, MSWD = 1.1, n=10) for a discordia anchored at the common ratio of 0.85 ± 0.04, determined by Roberts et al. (2017). To obtain the known intercept age of 254.4 ± 6.4 Ma for the WC-1 calcite, we applied a linear correction factor of 1.0031 to the measured ratios, which we also applied to all the samples analysed in the session, as is commonly done for carbonate U–Pb dating by LA-ICP-MS (e.g. Roberts et al., 2017; Drost et al., 2018; Kylander-Clark, 2020). All calculated dates are associated with two uncertainties: the first one includes the random uncertainties for each analysis (internal uncertainties on measured and ratios and reproducibility on repeated NIST614 analyses), while in the second one, systematic uncertainties (2.5 % uncertainty on the WC-1 age and 0.14 % and 0.11 % on the 235U and 238U decay constants, respectively; Jaffey et al., 1971) are propagated by quadratic addition.
The data obtained on the DBT calcite yielded a lower intercept date of 64.9 ± 2.2/2.8 Ma (95 % confidence level, MSWD = 2.9, n=12) for a discordia anchored at a common ratio of 0.74 ± 0.02, calculated based on isotope dilution and multi-collector ICP-MS analyses (Hill et al., 2016) (Fig. S1 in the Supplement). Because of its lower U abundance and younger age, the data obtained on the AUG-B6 calcite are less precise, yielding a concordia date of 40.8 ± 2.0/2.2 Ma (95 % confidence level, MSWD concordance + equivalence = 2.2, n=8) (Fig. S1), which is identical to a weighted average date of 41.6 ± 2.1/2.3 Ma (95 % confidence level, MSWD = 0.4, n=8). All results are available in Table S3.
Table 1LA-ICP-MS results for ALH 84001 carbonates.
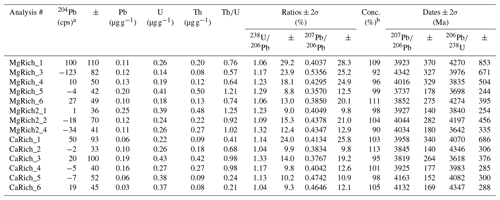
a Background-corrected 204Pb intensity, calculated using the measured 204(Pb+Hg) and 202Hg, and a of 0.22932.
b Concordance (100 × date date).
The carbonates analysed in ALH 84001 contain ca. 0.1–0.4 µg g−1 U and 0.1–0.5 µg g−1 Th (Table 1). When plotted in a Tera–Wasserburg vs. diagram, ALH 84001 carbonates yield a concordant date of 3941 ± 49/110 Ma (n=14, MSWD = 2.0; Fig. 2a), which is identical to a weighted mean date of 3967 ± 56/113 Ma (n=14, MSWD = 1.9; Fig. 2b). In detail, the Mg-rich and Ca-rich carbonate analyses yield concordia dates of 3890 ± 72 Ma (2σ, MSWD = 1.5, n=8) and 3995 ± 69 Ma (2σ, MSWD = 2.4, n=6), respectively; U–Pb dates for these two carbonate compositions are, therefore, indistinguishable when uncertainties are considered. Carbonate analyses plot on the concordia curve, indicating that they do not contain appreciable amounts of common Pb. This is consistent with the measured 204Pb intensities that are within error of 0 counts per second, although it is fair to point out that this observation is qualitative, considering the large uncertainties associated with the 202Hg, 204(Hg+Pb), and calculated 204Pb count rates (Tables 1 and S3). It is noteworthy that 202Hg intensities in ALH 84001 carbonates are about an order of magnitude higher than in the terrestrial carbonate standards (Table S3). This could indicate that martian carbonates contain higher Hg abundances than terrestrial carbonates. Alternatively, we think that this extra Hg likely originates from contamination of the ALH 84001 section by Au coating applied in the past for secondary ion mass spectrometry studies.
5.1 Comparison with previous ALH 84001 carbonate dating studies
In situ U–Pb dating of carbonates in ALH 84001 using LA-ICP-MS yields a concordia date of 3941 ± 49/110 Ma, which is identical to the carbonate step-leaching Rb-Sr isochron date of 3.94 ± 0.02 Ga (Borg et al., 1999; Beard et al., 2013) and the less precise isochron date of 4.045 ± 0.090 Ga (Borg et al., 1999). Before propagating the ± 2.5 % uncertainty associated with the age of the primary U–Pb reference carbonate WC-1 and the uncertainties associated with the 238U and 235U decay constants, the carbonate U–Pb concordia date is associated with a fairly precise 2σ uncertainty of ± 1.2 %, which increases to ± 2.8 % when all uncertainties are propagated. This suggests that in situ U–Pb dating of carbonates has the potential to yield precise dates, but it also highlights the need to reduce uncertainties on reference materials. Our LA-ICP-MS results also indicate that using a calcite primary reference material for correcting fractionation in Mg- and Fe-rich carbonate matrices, such as magnesite and ankerite, produce accurate dates (within the obtained uncertainties). A final point worth highlighting is the fact that carbonates in ALH 84001 do not contain appreciable amounts of common Pb, as indicated by their concordant U–Pb date of ca. 3.94 Ga. This is unusual, as most examples in terrestrial system carbonates do contain common Pb, incorporated during their crystallisation (e.g. Roberts et al., 2020). This observation suggests that the fluids from which ALH 84001 carbonates formed contained very little Pb.
5.2 Robustness of the carbonate U–Pb chronometer and further applications
Our in situ LA-ICP-MS analyses confirm that carbonates in ALH 84001 formed ca. 3.94 Ga ago and that the U–Pb chronometer in these carbonates has remained closed to any disturbance event since they formed. This is consistent with the suggestion that not much happened to ALH 84001 between 3.9 Ga and its launch from Mars 14 Ma ago (Treiman, 2021), and indicates that this latter event did not reset the carbonate U–Pb chronometer. From the evidence summarised in Sect. 1, Treiman (2021) concluded that minerals in ALH 84001, including the carbonates, have experienced neither temperatures in excess of ca. 25 ∘C nor exposure to any hydrous fluids since 3.9 Ga, leading to the conclusion that the climate of Mars has remained globally cold and dry since then. Any aqueous events occurring on Mars due to a globally warmer and wetter climate, therefore, took place before 3.9 Ga. Heating and aqueous alteration events due to meteoroid impacts could have occurred at any time, but these would have been strictly localised. Our U–Pb data back up the view that the carbonates in ALH 84001 have not experienced any alteration since they formed, supporting the conclusion that the progenitor material of ALH 84001 was not exposed to hydrous fluids or high temperature events for the last 3.9 Ga, suggesting a globally cold and dry Mars since then.
On the other hand, carbonates in terrestrial Archean samples are probably not the best suited for U–Pb dating, as most Archean formations would have been heated up to at least low greenschist metamorphic conditions and/or would have been affected by hydrothermal alteration, because the Earth is geologically active and harbours a complex hydrological cycle. On the other hand, the results of this study open up opportunities for dating old carbonates in samples that have had a relatively simple history post-carbonate formation. For example, volatile-rich carbonaceous chondrites (e.g. CI and CM chondrites) typically contain carbonates formed during fluid–rock interactions on their parent asteroids ca. 4563–4561 Ma-ago (e.g. Lee et al., 2014; Jilly-Rehak et al., 2017, and references therein), which is within 10 Myr of the formation of the first solids in the solar system. Bulk CI and CM chondrites contain ∼ 10 ng g−1 U (e.g. Braukmüller et al., 2018; Turner et al., 2021), a significant proportion of which is potentially hosted in labile phases such as carbonates (e.g. Burkhardt et al., 2019; Turner et al., 2021). As they make up ∼ 1 vol. %–2 vol. % of CM chondrites (e.g. Lee et al., 2014), these carbonates could host a few 100's ng g−1 U, which is similar to the U abundance in the ALH 84001 carbonates analysed here. After this phase of early hydrothermal alteration, during which carbonates formed, it is thought that not much happens to volatile-rich carbonaceous chondrites on their parent asteroids until they end up on the Earth as meteorite fragments. Carbonates in carbonaceous chondrites could thus be prime targets to further constrain the timing of hydrothermal alteration on volatile-rich asteroids using the U–Pb dating chronometer.
Raw LA-ICP-MS data and metadata are provided in the Supplement.
The supplement related to this article is available online at: https://doi.org/10.5194/gchron-4-683-2022-supplement.
ICL acquired and prepared the sample. RT designed the experiment, performed the analysis, and interpreted the results. RT prepared the manuscript with contributions from ICL.
The contact author has declared that neither of the authors has any competing interests.
Publisher's note: Copernicus Publications remains neutral with regard to jurisdictional claims in published maps and institutional affiliations.
We acknowledge the provision of the sample of ALH 84001 from the NASA Ancient Mars Meteorite Programme and funding from STFC and The University of Manchester. Many thanks to Nick Roberts for providing us with the WC-1 calcite, Benjamin Brigaud and Thomas Blaise for the AUG-B6 calcite, and Victor Polyak for the Duff Brown Tank calcite. We also thank Graham Edwards and an anonymous reviewer for their constructive suggestions.
This research has been supported by the Science and Technology Facilities Council (grant nos. ST/P005225/1 and ST/S002170/1).
This paper was edited by Brenhin Keller and reviewed by Graham Edwards and one anonymous referee.
Baker, J., Peate, D., Waight, T., and Meyzen, C.: Pb isotopic analysis of standards and samples using a 207Pb–204Pb double spike and thallium to correct for mass bias with a double-focusing MC-ICP-MS, Chem. Geol., 211, 275–303, 2004.
Bau, M., Romer, R. L., Lüders, V., and Beukes, N. J.: Pb, O, and C isotopes in silicified Mooidraai dolomite (Transvaal Supergroup, South Africa): implications for the composition of Paleoproterozoic seawater and `dating' the increase of oxygen in the Precambrian atmosphere, Earth Planet. Sc. Lett., 174, 43–57, 1999.
Beard, B. L., Ludois, J. M., Lapen, T. J., and Johnson, C. M.: Pre-4.0 billion year weathering on Mars constrained by Rb–Sr geochronology on meteorite ALH84001, Earth Planet. Sc. Lett., 361, 173–182, 2013.
Beaudoin, N., Lacombe, O., Roberts, N. M., and Koehn, D.: U-Pb dating of calcite veins reveals complex stress evolution and thrust sequence in the Bighorn Basin, Wyoming, USA, Geology, 46, 1015–1018, 2018.
Bertok, C., Barale, L., d'Atri, A., Martire, L., Piana, F., Rossetti, P., and Gerdes, A.: Unusual marbles in a non-metamorphic succession of the SW Alps (Valdieri, Italy) due to early Oligocene hydrothermal flow, Int. J. Earth Sci., 108, 693–712, 2019.
Borg, L. E., Connelly, J. N., Nyquist, L. E., Shih, C. Y., Wiesmann, H., and Reese, Y.: The age of the carbonates in martian meteorite ALH84001, Science, 286, 90–94, 1999.
Bouvier, A., Blichert-Toft, J., and Albarede, F.: Martian meteorite chronology and the evolution of the interior of Mars, Earth Planet. Sc. Lett., 280, 285–295, 2009.
Braukmüller, N., Wombacher, F., Hezel, D. C., Escoube, R., and Münker, C.: The chemical composition of carbonaceous chondrites: Implications for volatile element depletion, complementarity and alteration, Geochim. Cosmochim. Ac., 239, 17–48, 2018.
Bridges, J. C., Hicks, L. J., and Treiman, A. H.: Carbonates on Mars, in: Volatiles in the Martian Crust, edited by: Filiberto, J. and Schwenzer, S. P., Elsevier, Amsterdam, 89–118, https://doi.org/10.1016/B978-0-12-804191-8.00005-2, 2019.
Brigaud, B., Andrieu, S., Blaise, T., Haurine, F., and Barbarand, J.: Calcite uranium–lead geochronology applied to hardground lithification and sequence boundary dating, Sedimentology, 68, 168–195, 2021.
Burisch, M., Gerdes, A., Walter, B. F., Neumann, U., Fettel, M., and Markl, G.: Methane and the origin of five-element veins: mineralogy, age, fluid inclusion chemistry and ore forming processes in the Odenwald, SW Germany, Ore Geol. Rev., 81, 42–61, 2017.
Burisch, M., Walter, B. F., Gerdes, A., Lanz, M., and Markl, G.: Late-stage anhydrite-gypsum-siderite-dolomite-calcite assemblages record the transition from a deep to a shallow hydrothermal system in the Schwarzwald mining district, SW Germany, Geochim. Cosmochim. Ac., 223, 259–278, 2018.
Burkhardt, C., Dauphas, N., Hans, U., Bourdon, B., and Kleine, T.: Elemental and isotopic variability in solar system materials by mixing and processing of primordial disk reservoirs, Geochim. Cosmochim. Ac., 261, 145–170, 2019.
Cassata, W. S., Shuster, D. L., Renne, P. R., and Weiss, B. P.: Evidence for shock heating and constraints on martian surface temperatures revealed by thermochronometry of martian meteorites, Geochim. Cosmochim. Ac., 74, 6900–6920, 2010.
Coogan, L. A., Parrish, R. R., and Roberts, N. M.: Early hydrothermal carbon uptake by the upper oceanic crust: Insight from in situ U-Pb dating, Geology, 44, 147–150, 2016.
Corrigan, C. M. and Harvey, R. P.: Multi-generational carbonate assemblages in martian meteorite Allan Hills 84001: implications for nucleation, growth, and alteration, Meteorit. Planet. Sci., 39, 17–30, 2004.
del Real, P. G., Maher, K., Kluge, T., Bird, D. K., Brown, G. E., and John, C. M.: Clumped-isotope thermometry of magnesium carbonates in ultramafic rocks, Geochim. Cosmochim. Ac., 193, 222–250, 2016.
Drake, H., Roberts, N. M. W., Heim, C., Whitehouse, M. J., Siljeström, S., Kooijman, E., Broman, C., Ivarsson, M., and Åström, M. E.: Timing and origin of natural gas accumulation in the Siljan impact structure, Sweden, Nat. Commun., 10, 1–14, 2019.
Drake, H., Roberts, N. M., and Whitehouse, M. J.: Geochronology and Stable Isotope Analysis of Fracture-fill and Karst Mineralization Reveal Sub-Surface Paleo-Fluid Flow and Microbial Activity of the COSC-1 Borehole, Scandinavian Caledonides, Geosciences, 10, 56, https://doi.org/10.3390/geosciences10020056, 2020.
Drost, K., Chew, D., Petrus, J. A., Scholze, F., Woodhead, J. D., Schneider, J. W., and Harper, D. A.: An Image Mapping Approach to U-Pb LA-ICP-MS Carbonate Dating, and Applications to Direct Dating of Carbonate Sedimentation, Geochem. Geophy. Geosy., 19, 4631–4648, 2018.
Eugster, O., Weigel, A., and Polnau, E.: Ejection times of martian meteorites, Geochim. Cosmochim. Ac., 61, 2749–2757, 1997.
Fairey, B., Tsikos, H., Corfu, F., and Polteau, S.: U–Pb systematics in carbonates of the Postmasburg Group, Transvaal Supergroup, South Africa: primary versus metasomatic controls, Precambrian Res., 231, 194–205, 2013.
Godeau, N., Deschamps, P., Guihou, A., Leonide, P., Tendil, A., Gerdes, A., Hamelin, B., and Girard, J. P.: U-Pb dating of calcite cement and diagenetic history in microporous carbonate reservoirs: Case of the Urgonian Limestone, France, Geology, 46, 247–250, 2018.
Goodfellow, B. W., Viola, G., Bingen, B., Nuriel, P., and Kylander-Clark, A. R.: Palaeocene faulting in SE Sweden from U–Pb dating of slickenfibre calcite, Terra Nova, 29, 321–328, 2017.
Halevy, I., Fischer, W. W., and Eiler, J. M.: Carbonates in the martian meteorite Allan Hills 84001 formed at 18 ± 4 ∘C in a near-surface aqueous environment, P. Natl. Acad. Sci. USA, 108, 16895–16899, 2011.
Hansman, R. J., Albert, R., Gerdes, A., and Ring, U.: Absolute ages of multiple generations of brittle structures by U-Pb dating of calcite, Geology, 46, 207–210, 2018.
Hill, C. A., Polyak, V. J., Asmerom, Y., and Provencio, P.: Constraints on a Late Cretaceous uplift, denudation, and incision of the Grand Canyon region, southwestern Colorado Plateau, USA, from U-Pb dating of lacustrine limestone: U-Pb age of lacustrine limestone, Tectonics, 35, 896–906, 2016.
Holdsworth, R. E., McCaffrey, K. J. W., Dempsey, E., Roberts, N. M. W., Hardman, K., Morton, A., Feely, M., Hunt, J., Conway, A., and Robertson, A.: Natural fracture propping and earthquake-induced oil migration in fractured basement reservoirs, Geology, 47, 700–704, 2019.
Holland, G., Saxton, J., Lyon, I., and Turner, G.: Negative δ18O values in Allan Hills 84001 carbonate: possible evidence for water precipitation on Mars, Geochim. Cosmochim. Ac., 69, 1359–1370, 2005.
Jaffey A. H., Flynn, K. F., Glendenin, L. E., Bentley, W. C., and Essling, A. M.: Precision measurement of half-lives and specific activities of 235U and 238U, Phys. Rev. C, 4, 1889–1906, 1971.
Jilly-Rehak, C. E., Huss, G. R., and Nagashima, K.: 53Mn–53Cr radiometric dating of secondary carbonates in CR chondrites: Timescales for parent body aqueous alteration, Geochim. Cosmochim. Ac., 201, 224–244, 2017.
Jochum, K. P., Weis, U., Stoll, B., Kuzmin, D., Yang, Q., Raczek, I., Jacob, D. E., Stracke, A., Birbaum, K., Frick, D. A., Gunther, D., and Enzweiler, J.: Determination of reference values for NIST SRM 610–617 glasses following ISO guidelines, Geostand. Geoanal. Res., 35, 397–429, 2011.
Krähenbühl, U., Noll, K., Dobeli, M., Grambole, D., Herrmann, F., and Tobler, L.: Exposure of Allan Hills 84001 and other achondrites on the Antarctic Ice, Meteorit. Planet. Sci., 33, 665–670, 1998.
Kylander-Clark, A. R. C.: Expanding the limits of laser-ablation U–Pb calcite geochronology, Geochronology, 2, 343–354, https://doi.org/10.5194/gchron-2-343-2020, 2020.
Lapen, T., Righter, M., Brandon, A., Debaille, V., Beard, B., Shafer, J., and Peslier, A.: A younger age for ALH84001 and its geochemical link to shergottite sources in Mars, Science, 328, 347–351, 2010.
Lee, M. R., Lindgren, P., and Sofe, M. R.: Aragonite, breunnerite, calcite and dolomite in the CM carbonaceous chondrites: High fidelity recorders of progressive parent body aqueous alteration, Geochim. Cosmochim. Ac., 144, 126–156, 2014.
Li, Q., Parrish, R. R., Horstwood, M. S. A., and McArthur, J. M.: U–Pb dating of cements in Mesozoic ammonites, Chem. Geol., 376, 76–83, 2014.
MacDonald, J. M., Faithfull, J. W., Roberts, N. M. W., Davies, A. J., Holdsworth, C. M., Newton, M., Williamson, S., Boyce, A., and John, C. M.: Clumped-isotope palaeothermometry and LA-ICP-MS U–Pb dating of lava-pile hydrothermal calcite veins, Contrib. Mineral. Petr., 174, 63, https://doi.org/10.1007/s00410-019-1599-x, 2019.
Mittlefehldt, D. W.: ALH84001, a cumulate orthopyroxenite member of the Martian meteorite clan, Meteoritics, 29, 214–221, 1994.
Moorbath, S., Taylor, P. N., Orpen, J. L., Treloar, P., and Wilson, J. F.: First direct radiometric dating of Archaean stromatolitic limestone, Nature, 326, 865–867, 1987.
Mueller, M., Igbokwe, O. A., Walter, B., Pederson, C. L., Riechelmann, S., Richter, D. K., Albert, R., Gerdes, A., Buhl, D., Neuser, R. D., Bertotti, G., and Immenhauser, A.: Testing the preservation potential of early diagenetic dolomites as geochemical archives, Sedimentology, 67, 849–881, 2020.
Nicholson, S. L., Pike, A. W., Hosfield, R., Roberts, N. M. W., Sahy, D., Woodhead, J., Cheng, H., Edwards, R. L., Affolter, S., Leuenberger, M., and Burns, S. J.: Pluvial periods in Southern Arabia over the last 1.1 million-years, Quaternary Sci. Rev., 229, 106112, https://doi.org/10.1016/j.quascirev.2019.106112, 2020.
Nuriel, P., Weinberger, R., Kylander-Clark, A. R. C., Hacker, B. R., and Craddock, J. P.: The onset of the Dead Sea transform based on calcite age-strain analyses, Geology, 45, 587–590, 2017.
Nuriel, P., Craddock, J., Kylander-Clark, A. R., Uysal, T., Karabacak, V., Dirik, R. K., Hacker, B. R., and Weinberger, R.: Reactivation history of the North Anatolian fault zone based on calcite age-strain analyses, Geology, 47, 465–469, 2019.
Pagel, M., Bonifacie, M., Schneider, D. A., Gautheron, C., Brigaud, B., Calmels, D., Cros, A., Saint-Bezar, B., Landrein, P., Sutcliffe, C., Davis, D., and Chaduteau, C.: Improving paleohydrological and diagenetic reconstructions in calcite veins and breccia of a sedimentary basin by combining Δ47 temperature, δ18Owater and U-Pb age, Chem. Geol., 481, 1–17, 2018.
Parrish, R. R., Parrish, C. M., and Lasalle, S.: Vein calcite dating reveals Pyrenean orogen as cause of Paleogene deformation in southern England, J. Geol. Soc., 175, 425–442, 2018.
Paton, C., Hellstrom, J., Paul, B., Woodhead, J., and Hergt, J.: Iolite: Freeware for the visualisation and processing of mass spectrometric data, J. Anal. Atom. Spectrom., 26, 2508–2518, 2011.
Ray, J. S., Veizer, J., and Davis, W. J.: C, O, Sr and Pb isotope systematics of carbonate sequences of the Vindhyan Supergroup, India: age, diagenesis, correlations and implications for global events, Precambrian Res., 121, 103–140, 2003.
Ring, U. and Gerdes, A.: Kinematics of the Alpenrhein-Bodensee graben system in the Central Alps: Oligocene/Miocene transtension due to formation of the Western Alps arc, Tectonics, 35, 1367–1391, 2016.
Roberts, N. M. W. and Walker, R. J.: U-Pb geochronology of calcite-mineralized faults: Absolute timing of rift-related fault events on the northeast Atlantic margin, Geology, 44, 531–534, 2016.
Roberts, N. M. W., Rasbury, E. T., Parrish, R. R., Smith, C. J., Horstwood, M. S. A., and Condon, D. J.: A calcite reference material for LA-ICP-MS U-Pb geochronology, Geochem. Geophy., Geosy., 18, 2807–2814, 2017.
Roberts, N. M. W., Drost, K., Horstwood, M. S. A., Condon, D. J., Chew, D., Drake, H., Milodowski, A. E., McLean, N. M., Smye, A. J., Walker, R. J., Haslam, R., Hodson, K., Imber, J., Beaudoin, N., and Lee, J. K.: Laser ablation inductively coupled plasma mass spectrometry (LA-ICP-MS) U–Pb carbonate geochronology: strategies, progress, and limitations, Geochronology, 2, 33–61, https://doi.org/10.5194/gchron-2-33-2020, 2020.
Sarangi, S., Gopalan, K., and Kumar, S.: Pb–Pb age of earliest megascopic, eukaryotic alga bearing Rohtas Formation, Vindhyan Supergroup, India: implications for Precambrian atmospheric oxygen evolution, Precambrian Res., 132, 107–121, 2004.
Scardia, G., Parenti, F., Miggins, D. P., Gerdes, A., Araujo, A. G., and Neves, W. A.: Chronologic constraints on hominin dispersal outside Africa since 2.48 Ma from the Zarqa Valley, Jordan, Quaternary Sci. Rev., 219, 1–19, 2019.
Shaheen, R., Niles, P. B., Chong, K., Corrigan, C. M., and Thiemens, M. H.: Carbonate formation events in ALH 84001 trace the evolution of the martian atmosphere, P. Natl. Acad. Sci. USA, 112, 336–341, 2015.
Shuster, D. L. and Weiss, B. P.: Martian surface paleotemperatures from thermochronology of meteorites, Science, 309, 594–600, 2005.
Smeraglia, L., Aldega, L., Billi, A., Carminati, E., Di Fiore, F., Gerdes, A., Albert, R., Rossetti, F., and Vignaroli, G.: Development of an intra-wedge tectonic mélange by out-of-sequence thrusting, buttressing, and intraformational rheological contrast, Mt. Massico ridge, Apennines, Italy, Tectonics, 38, 1223–1249, 2019.
Treiman, A. H.: Uninhabitable and Potentially Habitable Environments on Mars: Evidence from Meteorite ALH 84001, Astrobiology, 21, 940–953, 2021.
Turner, S., McGee, L., Humayun, M., Creech, J., and Zanda, B.: Carbonaceous chondrite meteorites experienced fluid flow within the past million years, Science, 371, 164–167, 2021.
Vermeesch, P.: IsoplotR: a free and open toolbox for geochronology, Geosci. Front., 9, 1479–1493, 2018.
Villa, I. M., De Bievre, P., Holden, N. E., and Renne, P. R.: IUPAC–IUGS recommendation on the half-life of 87Rb, Geochim. Cosmochim. Ac., 164, 382–385, 2015.
Walter, B. F., Gerdes, A., Kleinhanns, I. C., Dunkl, I., von Eynatten, H., Kreissl, S., and Markl, G.: The connection between hydrothermal fluids, mineralization, tectonics and magmatism in a continental rift setting: Fluorite Sm-Nd and hematite and carbonates U-Pb geochronology from the Rhinegraben in SW Germany, Geochim. Cosmochim. Ac., 240, 11–42, 2018.