the Creative Commons Attribution 4.0 License.
the Creative Commons Attribution 4.0 License.
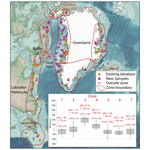
The marine reservoir age of Greenland coastal waters
Christof Pearce
Karen Søby Özdemir
Ronja Forchhammer Mathiasen
Henrieka Detlef
Jesper Olsen
Knowledge of the marine reservoir age is fundamental for creating reliable chronologies of marine sediment archives based on radiocarbon dating. This age difference between the 14C age of a marine sample and that of its contemporaneous atmosphere is dependent on several factors (among others, ocean circulation, water mass distribution, terrestrial runoff, upwelling, and sea-ice cover) and is therefore spatially heterogeneous. Anthropogenic influence on the global isotopic carbon system, mostly through atmospheric nuclear tests, has complicated the determination of the regional reservoir age correction ΔR, which therefore can only be measured in historic samples of known age. In this study we expand on the few existing measurements of ΔR for the coastal waters around Greenland, by adding 92 new radiocarbon dates on mollusks from museum collections. All studied mollusk samples were collected during historic expeditions of the late 19th and early 20th centuries, and besides coastal sites around Greenland, the new measurements also include localities from the western Labrador Sea, Baffin Bay, and the Iceland Sea. Together with existing measurements, the new results are used to calculate average ΔR values for different regions around Greenland, all in relation to Marine20, the most recent marine radiocarbon calibration curve. To support further discussions and comparison with previous datasets, we use the term ΔR13, where the suffix 13 refers to the previous calibration curve Marine13. Our study explores the links between the marine reservoir age and oceanography, sea-ice cover, water depth, mollusk feeding habits, and the presence of carbonate bedrock. Although we provide regional averages, we encourage people to consult the full catalogue of measurements and determine a suitable ΔR for each case individually, based on the exact location including water depth. Despite this significant expansion of the regional reservoir age database around Greenland, data from the northern coast, directly bordering the Arctic Ocean, remain missing.
- Article
(4391 KB) - Full-text XML
-
Supplement
(6820 KB) - BibTeX
- EndNote
1.1 Radiocarbon dating of marine samples
The most common method for obtaining ages of sediments younger than 50 000 years is radiocarbon dating. This method relies on the uptake of radiocarbon (14C) by plants and animals in equilibrium with their environment and, following the death of the organism, the subsequent disconnection between them. The 14C in the sample then decays to 14N through beta emission with a half-life of 5700 years (Audi et al., 2003). Provided the carbon within the sample has remained a closed system and the initial 14C activity is known, the remaining 14C concentration of the sample can be used to calculate its age. To account for the temporal variations in 14C concentration in the atmosphere and oceans, radiocarbon dating relies on calibration data, which enable the conversion to calibrated ages (Heaton et al., 2021). The most recent such calibration datasets are IntCal20 (Reimer et al., 2020), SHCal20 (Hogg et al., 2020), and Marine20 (Heaton et al., 2020), for Northern Hemisphere atmospheric, Southern Hemisphere atmospheric, and marine samples, respectively. For the purpose of radiocarbon dating, the atmosphere is considered to be well-mixed, with a uniform global distribution of 14C content in each hemisphere. In contrast, the ocean is a more heterogeneous environment due to spatially varying stratification and upwelling intensity and the overall relatively slow mixing of water masses. The intermediate and deep ocean are typically much more depleted in 14C compared to the surface ocean, which can lead to differences in radiocarbon age of centuries to millennia across the water column (Broecker et al., 1960; Matsumoto, 2007). The age of the surface ocean is thus influenced by different carbon reservoirs each with their own 14C content, a phenomenon called the reservoir effect (Stuiver and Braziunas, 1993). The reservoir age is time-dependent, termed R(t), and is defined as the difference between the radiocarbon age of a sample from within the reservoir and the age of the contemporaneous atmosphere (Stuiver et al., 1986). In the Marine20 calibration dataset, the modeled global average marine reservoir age is approximately 550 years (Heaton et al., 2020), but this value varies spatially due to differences in ocean circulation, upwelling, runoff, and gas exchange with the atmosphere. When calibrating marine radiocarbon dates, this spatial heterogeneity is accounted for by applying a regional reservoir age correction, ΔR (Reimer and Reimer, 2001; Stuiver et al., 1986), which is defined as the contemporaneous difference between the 14C age of the reservoir and the global marine calibration curve, e.g., Marine20 or Marine13.
In principle, ΔR could be calculated from the radiocarbon age of a modern marine sample, but anthropogenic contamination has complicated this approach on recent samples (Mangerud, 1972). Increased input of 14C from bomb testing (Hesshaimer et al., 1994) has made it impossible to measure the modern reservoir age of the surface ocean. To determine ΔR, we therefore rely on samples that predate the contamination (ca. 1950 CE) and where the age of the sample is known or independently determined through either tephrochronology (Pearce et al., 2017; Austin et al., 1995; Olsen et al., 2014), U–Th dating of corals (Hua et al., 2015), or paired marine–terrestrial dating (Ascough et al., 2005). The most common approach based on recent samples of known age is the use of museum collections where typically the exact locality, date, and other collection details are available and reliable. Several hundred different studies have been made to study the local reservoir age, and an overview of all shallow-water (<75 m) samples is provided online at The Marine Reservoir Database (Reimer and Reimer, 2001). This database is continuously updated and maintained, and currently more than 1000 data entries for the regional reservoir correction are listed, together with relevant metadata. The coverage is global but not evenly distributed geographically, and some regions (e.g., western Africa, northern Siberia, parts of Greenland) are represented by only very few sample stations (Alves et al., 2018; Reimer and Reimer, 2001).
1.2 The Marine20 calibration curve
The publication of the most recent marine radiocarbon calibration curve Marine20 (Heaton et al., 2020) introduced a new global average for the marine reservoir age compared to previous such datasets, including its predecessor Marine13 (Reimer et al., 2013). The globally averaged value of the reservoir age in the Marine20 model is significantly higher (about 155 years) compared to Marine13, which made it necessary to recalculate ΔR values so they could be used with the new Marine20 curve (Heaton et al., 2020). This has led to some confusion, however, since the paleoceanography community had gotten used to referring to regional reservoir correction values relative to the Marine13 or previous calibration curves (see also discussion in Pieńkowski et al., 2022). The calibration of a radiocarbon date using the Marine20 curve but corrected by an old (pre-2020) ΔR value simply results in the wrong final calibrated age, so it is crucial to use the appropriate updated ΔR value for the new curve. Examples of circum-Greenland studies where Marine20 was used, while the ages were corrected by an outdated ΔR value, are plenty (Olsen et al., 2022; Devendra et al., 2022; Sha et al., 2022; Peral et al., 2022; Allaart et al., 2021), and this has unfortunately led to errors in the final age models. To simplify the discussion and to enable us to cite previously used regional reservoir age correction values, we use the term ΔRxx where xx stands for the publication year of calibration curve. If no suffix is included, the term ΔR is here meant relative to the newest calibration curve Marine20. As an example, in Disko Bay, a regional reservoir correction of 140 ± 35 14C years based on measurements of pre-bomb Astarte mollusks (McNeely et al., 2006; Lloyd et al., 2011) has been used in multiple paleoceanographic studies (Moros et al., 2016; Krawczyk et al., 2017; Li et al., 2017; Ouellet-Bernier et al., 2014). From here onwards, we would cite such values as ΔR13 = 140 ± 35 14C years, here referring to the Marine13 curve. In this case of Disko Bay, the new correction value relative to Marine20 based on the same two mollusk samples is ΔR = 20 ± 76 14C years (Reimer and Reimer, 2001). Thus, although the total reservoir age R has not changed, the regional reservoir correction ΔR is different because of the different calibration curves used.
1.3 The Marine20 curve in polar regions
The most recent marine calibration curve update (Marine20) made it explicit that the calibration curve does not apply for the calibration of samples from polar regions (Heaton et al., 2020). This is, of course, a particular challenge for studies focusing on Greenland. The main reason to avoid Marine20 outside latitudes from 40∘ S–40∘ N is the variability in air–sea gas exchange due to changes in sea-ice cover and other local changes in surface water 14C concentrations in these cold regions. Especially in glacial periods, extensive sea-ice cover would reduce ventilation and can lead to significant increases in the local reservoir age of over 1000 years compared to modern values (Heaton et al., 2020). This limitation of using radiocarbon dating of marine samples from high latitudes is, however, not unique to the latest Marine20 dataset; it has been true for previous calibration curves as well, although not as explicitly mentioned. Simple box models have shown a consistent link between sea-ice cover and the radiocarbon reservoir age (Bard et al., 1994), and high-resolution studies using tephrochronology coupled with radiocarbon dates have documented North Atlantic reservoir ages of >2000 years during deep stadials of MIS3 (Olsen et al., 2014). Despite the advice against using Marine20 in polar regions, it has already been used extensively for this purpose since its publication (Pados-Dibattista et al., 2022; Glueder et al., 2022; Hansen et al., 2022; El bani Altuna et al., 2021; Brouard et al., 2021; Stevenard et al., 2022; Davies et al., 2022; Jackson et al., 2022). Since the publication of Marine20, a more recent paper by the same main authors has now been published and clarifies this issue. Heaton et al. (2023) state that their warning about using the marine calibration curve in polar regions also applies to previous versions and is not something new and unique to Marine20. The latest advice is now to use, with caution, the Marine20 calibration curve for polar samples of Holocene age (interglacial, non-14C-depleted surface ocean) and to use it as a lower bound for calibration samples from glacial periods during which the surface oceans may be more depleted (Heaton et al., 2023). This additional challenge with the calibration of polar marine radiocarbon dates emphasizes the importance of studies such as the one presented here, aiming to expand the knowledge of spatial variability in ΔR in these regions.
1.4 The marine reservoir age around Greenland
As anywhere else in the marine realm, the regional radiocarbon reservoir age around Greenland is influenced by ocean currents, terrestrial runoff, mixing of water masses of different ages, and variable exchange between the surface ocean and atmosphere. In the Arctic, the latter is heavily influenced by the presence of sea ice, which may inhibit mixing between surface waters and the overlying air. Greenland has perennial sea-ice cover along its northern coastline, while the southern coast typically experiences year-round ice-free conditions. These contrasting sea-ice conditions create a pattern of older surface waters along the Arctic Ocean and younger waters at the lower-latitude coastal waters facing the North Atlantic Ocean. In the surface ocean, east Greenland is characterized by Polar Water flowing out from the Arctic Ocean through the Fram Strait with the East Greenland Current. Similarly, northwest Greenland receives Polar Water coming through the Nares Strait and the Canadian Arctic Archipelago, also containing a larger share of older Pacific Water, originating from the Bering Strait (Jones et al., 2003). This pattern is further complicated, however, by the presence of well-ventilated, and thus younger, Atlantic waters that occupy the subsurface waters. Atlantic water is known to reach the Greenlandic coast in many locations, typically driven by the bathymetry, e.g., where deep glacially formed cross-shelf troughs allow this subsurface water connection (Schaffer et al., 2020; Sutherland et al., 2013; Millan et al., 2018). The perceived reservoir age correction ΔR as measured in certain organisms may also be impacted by the presence of carbonate bedrock in the area. This has a particular influence on deposit feeders, which may take up more old dissolved carbonate than filter-feeding mollusks (Mangerud et al., 2006; England et al., 2013; Forman and Polyak, 1997).
Existing measurements of regional marine reservoir age ΔR values from coastal waters of Greenland are relatively sparse and highly clustered around a few localities such as Disko Bay and Scoresby Sound (Hjort, 1973; McNeely et al., 2006; Tauber and Funder, 1975). In the north, no data at all exist above latitudes of 77 and 79∘ N, in the east and west Greenland, respectively (Reimer and Reimer, 2001). In the south, between 68∘ N and down to Greenland's southernmost point at Cape Farewell, only four measurements exist and are almost exclusively on mammals (polar bears and humans), the feeding area of which and marine fraction of whose diet is not exactly known (Olsson, 1980). This heterogeneously spaced dataset has allowed different studies to use various values for the regional reservoir correction. In studies of Holocene paleoceanography offshore Greenland, the most commonly used value for the reservoir age correction (prior to publication of Marine20), is ΔR13 = 0 14C years, depending on the year of publication either given as ΔR04, ΔR09, or ΔR13 (Knudsen et al., 2008; Levac et al., 2001; Jensen et al., 2004; Lassen et al., 2004; Nørgaard-Pedersen and Mikkelsen, 2009; Andresen et al., 2011; Seidenkrantz et al., 2008; Lloyd, 2006; Moros et al., 2006; Møller et al., 2006; Andrews and Jennings, 2014; Andresen et al., 2013). In Disko Bay, west Greenland, Lloyd et al. (2011) argued for ΔR09 = 140 ± 30 14C years, which was used in subsequent studies close to the study site (Moros et al., 2016; Krawczyk et al., 2017; Perner et al., 2013; Ouellet-Bernier et al., 2014; Li et al., 2017) as well as the wider region all the way to Sisimiut in the south (Erbs-Hansen et al., 2013) and Upernavik in the north (Hansen et al., 2020). In east Greenland, some studies have differentiated between sites north and south of the Denmark Strait. Jennings et al. (2011) used ΔR09 = 0 14C years on the shelf south of Denmark Strait based on the occurrence of several tephra layers and ΔR09 = 149 ± 99 14C years north of Denmark Strait, arguing for a stronger influence of older Polar Water at higher latitudes. A recurring argument for using a ΔR value of 0 14C years is the presence of young Atlantic waters in the subsurface corresponding to the sediment coring location (Andresen et al., 2013), while others have suggested that using the ΔR value of 0 14C years can be regarded as a minimum and therefore calibrated ages may be too old (Andrews and Jennings, 2014).
1.5 Aim of this study
To allow the determination of leads and lags in the climate system over long timescales, there is a need for robust correlations between geological archives from different environments. These correlations between marine, terrestrial, and ice-core records rely on independent, accurate and precise determinations of the age of sediment layers. In the marine environment, one of the main sources of errors in chronology is knowledge of the regional marine reservoir age correction (Alves et al., 2018). The spatial and temporal heterogeneity of this parameter requires local calibrations, and the present understanding of the modern ΔR values around Greenland is based on a relatively small number of samples. Besides the low number of available measurements of ΔR, a regional synthesis of the data is lacking for Greenland. Several such investigations exist for neighboring regions, including Arctic Canada (Coulthard et al., 2010; McNeely et al., 2006; Pieńkowski et al., 2022) and the northeastern North Atlantic (Mangerud et al., 2006). The aim of this study is to improve the current estimates of the regional reservoir age around Greenland and to summarize new and existing data into regional recommendations for use with the latest Marine20 calibration curve.
2.1 Museum sampling
Mollusk samples were collected from the zoology divisions of the Swedish Museum of Natural History (SMNH) in Stockholm, Sweden, and the Natural History Museum of Denmark (NHMD) in Copenhagen, Denmark. At the SMNH, a combination of a digital database and written catalogues was used to identify potential samples before sampling from the collections. At the NHMD, a lack of such catalogues meant that all samples were selected and sampled directly by visiting the sample storage facilities and manual inspection of labeled sample containers. In both museums, for selecting suitable mollusk samples, the following criteria were considered:
-
geographic location listed by coordinates (descriptive locations were excluded);
-
location in circum-Greenland waters in the broadest sense (including the Greenland Sea, North Atlantic Ocean, Labrador Sea, Baffin Bay);
-
known year of sampling and age no younger than 1950 CE;
-
sufficient material available, so at least one intact specimen could be left in the museum collection.
A total of 92 samples were included in this study from coastal and shelf waters of Newfoundland, Labrador, Baffin Island, Greenland, and a few open-ocean localities in the Labrador Sea and Greenland Sea (Fig. 1). The collection year of the samples ranges between 1865 and 1931 CE, so well before the introduction of bomb-derived 14C into the global carbon cycle (Hesshaimer et al., 1994). The samples were retrieved during various historic expeditions (Table S1 in the Supplement), with most specimens collected during the following four sampling campaigns: Ellis Nillson on the Scottish whaling ship Eclipse in 1894 to the northern part of Baffin Bay, Fredrik von Otter on the Ingegerd and Gladan Expedition of 1871 to the Labrador Sea and Baffin Bay, Alfred Gabriel Nathorst on the Antarctica to east Greenland in 1899, and Eigil Riis-Carstensen on the Godthåb Expedition of 1928 to the Labrador Sea and Baffin Bay (Liljequist, 1993; Nathorst, 1900; Riis-Carstensen, 1929).
Most samples (n=75) were taken from the so-called wet collections, i.e., stored in ethanol, but some dry samples (n=17) from the SMNH were included in this study as well. The dry samples were not studied in detail for signs of exposure or reworking, such as color changes, or remains of soft tissue or any other evidence of age of death before collection. In all wet samples, mollusk soft tissue was present before further processing, ensuring the specimens were alive at the time of sampling. Specimens were transferred to plastic sample containers and transported to Aarhus University for further processing.
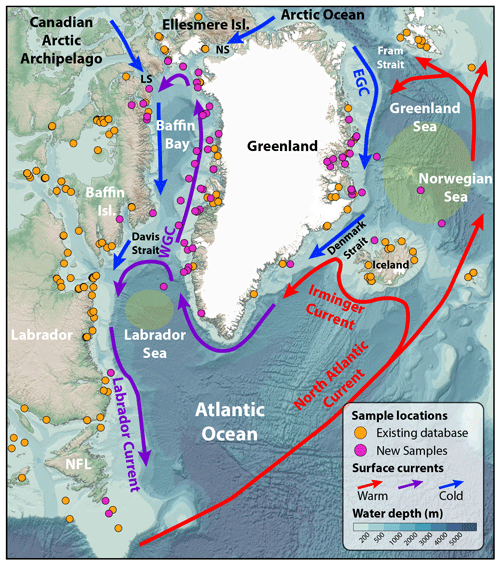
Figure 1Map of Greenland and the northern North Atlantic Ocean, with ocean currents and locations of all samples analyzed in this study together with those of the existing ΔR database (Reimer and Reimer, 2001). Areas of deep convection in the Labrador Sea and north of Iceland are shaded light green. LS: Lancaster Sound; NS: Nares Strait; NFL: Newfoundland; WGC: West Greenland Current; EGC: East Greenland Current. Bathymetry is from GEBCO (GEBCO Bathymetric Compilation Group, 2022).
2.2 Laboratory pre-treatment and 14C dating
Wet samples were placed in a drying oven at 40 ∘C for several days to remove any ethanol from the shell, before any remaining dried-up soft tissue was removed from the shell. Approximately 10–15 mg of carbonate was removed from the outer, youngest part of the shell for further treatment. As some Arctic mollusks are known to have a long lifespan of decades, even up to centuries (Wanamaker et al., 2008; Selin, 2010), this sampling approach avoids the possible incorporation of older carbonate. In case of very small mollusk samples, the entire shell was used (smallest sample 5.7 mg). To remove any surface contamination, the shells were placed in an HCl solution (1 M) for 2 h until 10 %–20 % of the carbonate was dissolved. During this step, the samples were carefully shaken a few times to ensure the acid could access the entire sample. After acid treatment the samples were carefully rinsed three times with Milli-Q® water. Each rinsing was done with its own glass and pipette, which were examined after the rinse to ensure that none of the sample was lost in the process. A small amount of water was left to lower the risk of flushing part of the sample. The samples were dried overnight to remove the excess water and then weighed. The remaining carbonate was subsequently converted to graphite and 14C dated using the HVE 1MV tandetron accelerator mass spectrometry (AMS) system at the Aarhus AMS Centre (Olsen et al., 2017). 14C dates are reported as uncalibrated 14C ages BP normalized to −25 ‰ according to international convention using online ratios (Stuiver and Polach, 1977).
2.3 Calculation of reservoir age per sample
The local reservoir age correction ΔR was calculated for each sample as the difference between the measured radiocarbon age of the sample and the radiocarbon age of the Marine20 calibration curve (Heaton et al., 2020) corresponding to the year of collection. To avoid errors and ensure reproducibility, all calculations were performed using the online program deltar (Reimer and Reimer, 2017) with the input of the measured radiocarbon age and associated uncertainty, and the year of sample collection, i.e., the independently known calendar age. The uncertainty in ΔR equals the uncertainty in the radiocarbon date, which therefore does not take into account the uncertainty in the marine curve (Reimer and Reimer, 2017).
2.4 Geographical zonation
To summarize the results and provide broad, regional estimates of the reservoir age corrections around Greenland, the study area was divided into seven zones, based on prevailing ocean currents and water masses; see Fig. 2. The zone boundaries are broadly consistent but not exactly the same as in previous ΔR compilation studies in the area (McNeely et al., 2006; Coulthard et al., 2010; Pieńkowski et al., 2022). Zone 1 covers the shelf seas south of Newfoundland, where there is a mixed influence of Atlantic Water coming from the North Atlantic Current in the south and colder waters from the north via the Labrador Current (Fig. 1). Zone 2 includes the coastal waters of the western Labrador Sea from northern Newfoundland in the south, along the Labrador Peninsula, to southern Baffin Island in the north (Fig. 2). These waters are predominantly influenced by the Labrador Current (Fig. 1), which includes a mixture of cold polar waters coming from the north and Atlantic Water originating from the West Greenland Current as it branches across the Davis Strait (Fig. 1). Zone 3 covers the northwestern part of Baffin Bay and represents a cluster of samples south of Lancaster Sound (Fig. 2). It is characterized by outflow of Arctic Waters through the Canadian Arctic Archipelago and Nares Strait (Fig. 1). Zone 4 includes Jones Sound south of Ellesmere Island and Smith Sound at the southern end of Nares Strait (Fig. 2) and, similarly to Zone 3, is predominantly influenced by Artic Water. Zone 5 encompasses the northwestern coast of Greenland, from the Davis Strait in the south to Nares Strait in the north (Fig. 2). It is dominated by waters of the West Greenland Current over the shelf (Fig. 1), while the more coastal inner fjord location will be impacted by outflow from the Greenland Ice Sheet as well. Zone 6 stretches all along the coast and shelf of southern Greenland. Its boundaries with Zone 7 and Zone 5 are set where the Irminger Current merges with the East Greenland Current and where the West Greenland Current branches off across the Davis Strait, respectively (Fig. 1). Finally, Zone 7 covers the coastal waters of northeast Greenland, including several sites inside deep fjord systems (Fig. 2). This region is dominated by the East Greenland Current carrying polar surface waters from the Arctic Ocean through the Fram Strait, underlain by recirculated warmer Atlantic Water at water depths >100 m (Fig. 1). Five samples that were analyzed in this study fell outside of the zonation: three from deep sites in the Greenland Sea and Norwegian Sea, one from northern Iceland, and one from a deep site in the Labrador Sea (Fig. 2).
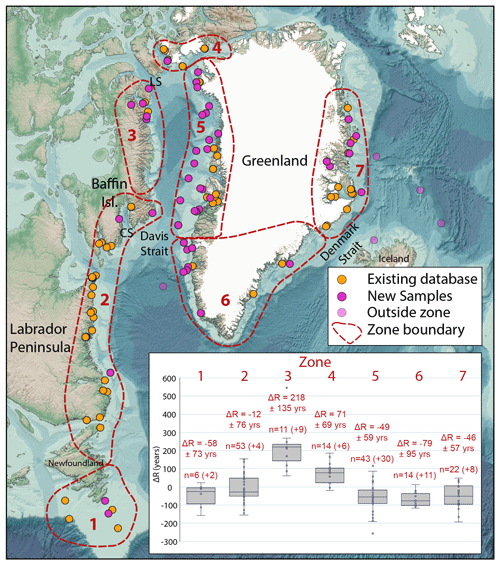
Figure 2Map of study area with boundaries of geographic zones and locations of all samples used for calculations (wet collection only). The insert shows zonal average ΔR values, based on all data points except the four that fall outside of the zone boundaries in the Greenland, Iceland, and Norwegian seas. The box-and-whisker charts show the interquartile range (box), the minimum and maximum values (whiskers), and the median value (midline in box). The values shown in red for each zone are the weighted mean values and uncertainty as described in the methods and also listed in Table 1. The number of data points is given for each zone, including the number of new values obtained in this study, listed in brackets. LS: Lancaster Sound; CS: Cumberland Sound. Bathymetry is from GEBCO (GEBCO Bathymetric Compilation Group, 2022).
2.5 Regional mean reservoir age correction estimates
To provide an average value and uncertainty for each of the regions, we followed the standard methodology as also applied in the Marine Reservoir Age Database (Reimer and Reimer, 2001) to calculate weighted means while incorporating the uncertainty in the original data. For each zone we calculate the ΔR as the weighted mean value using (Bevington and Robinson, 2002):
where ΔRi and σi are the mean value and uncertainty in calculated local reservoir age offset of sample i. The uncertainty in the weighted mean ΔR values is calculated as
where the subscript w indicates that the uncertainty is calculated using the uncertainty in each ΔRi. Further we calculated the standard deviation as
where n is number of samples and ΔRw is the regional weighted mean ΔR value.
No form of outlier analyses was performed and all available data, both previously published and new results, were included in the zonal mean values. To account for the larger than statistically expected variability in some of the ΔRw values, we take the maximum value of σw or σSD as the uncertainty on the ΔRw values.
3.1 Wet vs. dry museum mollusk collections
All dry samples analyzed in this study (n=17) are from the same region along the east Greenland coast between 70 and 75∘ N (Fig. 2). Mollusks stored in ethanol were analyzed from the same region, which allows comparison between the two samples sets based on their preservation. Although some radiocarbon dates indicate compatible ΔR values, there is a clear difference between the two datasets, with the dry samples being significantly older than the wet (Fig. S2 in the Supplement). At a single location, a dry sample (no. 83, Table S1) returned ΔR = 1141 ± 27 14C years, which is > 1000 years older than others from the same site (Fig. S2). There is no certainty that the dry samples were collected alive, and they are therefore considered unreliable for the purpose of determining the local marine reservoir age. All dry samples are excluded from further discussion in this paper, and all reporting of results from here onwards is of wet-preserved samples only.
3.2 Results' summary
For the entire study area of coastal Greenland, including the Labrador Sea and Baffin Bay, the newly obtained values for ΔR range from −172 to 546 14C years, with a median value of ΔR = −39 14C years. The geographical range of the samples reaches from 46∘ N south of Newfoundland to approximately 76 and 77∘ N, on the eastern and western Greenlandic shelf, respectively (Fig. 1). The majority of new samples are from west Greenland, originating mostly from the 1928 Godthåb Expedition (Riis-Carstensen, 1929). Whereas samples in the online marine reservoir correction database are generally restricted to shallow water depths <75 m (Reimer and Reimer, 2001), our new samples represent the broader coastal shelf settings and expand to water depths of typically several hundred meters (Fig. 1, Table S1). The full depth scale of the newly dated samples ranges from a black mussel collected on the beach in Cumberland Sound (Musculus niger, sample no. 5, Table S1) to a gastropod collected from 2750 m water depth (m wd) in the northern central Labrador Sea (Buccinum abyssorum, sample no. 61, Table S1).
3.3 Reservoir age offsets per region
The new data for the regional marine reservoir age offsets (ΔR) are here reported for each region, as defined in the “Materials and methods” section and illustrated in Fig. 2. The results of the 75 newly dated mollusk samples from wet collections are combined with previously existing data from the marine reservoir correction database (Reimer and Reimer, 2001). The results per zone are summarized in Table 1. Although there is overlap between the new ΔR values from the entire study area, there are also clear differences between specific zones. The weighted mean ΔR values for all zones fall between 0 and −100 14C years, except for zones 3 and 4, where the ΔR values are higher. The highest values occur in Zone 3, south of Lancaster Sound (Fig. 2), with a mean ΔR value for this zone of 218 14C years. The mean values for Greenland coastal waters from all three zones (east, west, and south) all overlap within the calculated uncertainties (Table 1).
Table 1Summary of regional ΔR values. Total samples include existing and new samples from this study (number listed in parentheses). Range, weighted means, and uncertainties are calculated on the full dataset. Zone numbers refer to Fig. 2.
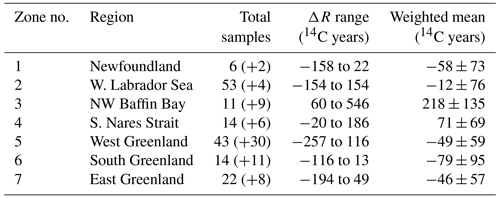
Five new samples fall outside the geographical zone boundaries: no. 65, no. 66, and no. 75 from the Norwegian Sea and Greenland Sea; no. 64 from the shelf north of Iceland; and no. 61 from the central Labrador Sea. These five are mostly from deeper sites (> 1500 m wd), except for sample no. 64 which is from 388 m wd (Fig. S1, Table S1). All these deep samples returned relatively young ages, i.e., ΔR < 0 years, including the deepest sample (no. 61 from 2750 m wd), which returned one of the lowest values of ΔR in the entire dataset (ΔR = −149 ± 27 14C years).
The new results obtained in this study are overall in good agreement with previously existing measurements of the reservoir age offsets around Greenland and eastern Canada. There is significant overlap between existing and new measurements, and therefore this expansion adds to the reliability of regional estimates, but the new data also include many localities for which previously no information was available. The new measurements mostly come from shallow coastal locations but also include many sites on the shelf with water depths >100 m. This spatial expansion of available data is key for paleoceanographic studies as they are often based on sediment cores from deeper shelf locations where deglacial–Holocene sediments are dated using benthic organisms (e.g., mollusks or benthic foraminifera). The discussion presented here includes the relation of ΔR to water mass, water depth, sea-ice cover, and local variability within small areas, but it is not exhaustive, and the reader is encouraged to consult the complete tables of results in the Supplement to investigate specific cases.
4.1 Spatial patterns and ocean circulation
The overall results as shown in Fig. 2 are consistent with the ocean circulation patterns around Greenland, but there are also some notable observations. As expected, the lowest ΔR values are found in regions where Atlantic Water prevails: Zone 1 south of Newfoundland and Zone 6 in southern Greenland, along the path of the Irminger and West Greenland Current (Fig. 1). The oldest waters or highest ΔR values are found along Baffin Island and in the northernmost part of Baffin Bay (Fig. 2: zones 3 and 4), a region influenced by Arctic Waters coming through the Canadian Arctic (Coulthard et al., 2010). The age of the Arctic outflow is likely dependent on the water depth, with older waters of Pacific origin in the surface and younger Atlantic waters below. This depth-dependent reservoir age in the Arctic Ocean was also found by a study from the Chukchi Sea using tephrochronology to constrain the reservoir age during the Late Holocene (West et al., 2022). The waters in Zone 2 are not only influenced by Arctic outflow but also by the presence of carbonate in local bedrock, which may further explain older radiocarbon ages (England et al., 2013). For the coastal areas surrounding Baffin Bay, there is overlap with another recent reservoir age compilation study focusing on the Canadian Arctic (Pieńkowski et al., 2022). The results are mostly compatible, as both studies show a pattern of old values along the Canadian margin and younger waters along the west Greenland coast. The zonal mean ΔR values are, however, not exactly the same, since this study adds 45 new radiocarbon measurements for Baffin Bay and both studies also use a slightly different geographical zonation (Pieńkowski et al., 2022). In between the zones with mostly Atlantic versus Arctic and Pacific Water influence lie Zone 2 and Zone 5, and they show intermediate values, likely the result of mixing between the two endmembers. This is seen in the high variance in the ΔR values in these zones 2 and 5, which also cover a large latitudinal range (Fig. 2). Along east Greenland, remarkably, there is no major change in local reservoir age across the Denmark Strait, which was hypothesized in other studies to be caused by the influence of the Irminger Current (Jennings et al., 2011). The calculated ΔR values for zones 6 and 7 (Fig. 2), respectively, south and north of the Denmark Strait (approx. 67∘ N), are both well below zero, and the calculated mean values are not significantly different (Table 1). There is a larger variance in the ΔR values in Zone 7, however, but this is likely caused by the larger sample size and by the inclusion of many sites inside fjords in this zone (Fig. 2). Near-coastal sites inside fjords can also be influenced by terrestrial runoff which can modify the 14C age of waters on a very local scale.
4.2 Water depth
By definition, the marine reservoir offset is only valid for the surface ocean, i.e., the upper part of the water column that is well-mixed, with the maximum depth set to 75 m (Stuiver et al., 1986). This same water depth cutoff value of 75 m is used in the online marine reservoir correction database at http://calib.org/marine (last access: 11 December 2023) (Reimer and Reimer, 2017), although some exceptions have been made and data from deeper sites outside the surface mixed layer were included in the database (Lougheed et al., 2013). The publication of the latest marine calibration curve, Marine20, mentions a water depth of 100 m for the surface ocean box in the model, thus slightly extending the depth range for the definition of the surface ocean in reservoir age calculations (Heaton et al., 2020). However, despite this policy of restricting the ΔR database to the upper 75–100 m of the surface ocean, the marine calibration curves are routinely used in paleoceanography on sediment core samples from well outside of this depth range. In fjords or coastal shelf environments, water depths can easily reach several hundred meters, but planktic foraminifera or other calcifying surface ocean dwellers are often absent. The standard approach here is to resort to dating benthic organisms such as benthic foraminifera or mollusks and apply the local reservoir correction ΔR, which is originally intended for the surface ocean only. Even for deep-ocean sites where sediment cores are dated using planktic foraminifera one could argue that their habitat range is not restricted to the upper 75 m of the water column, but they are often found below that, up to several hundred meters water depth (Kimoto, 2015). This current study and expansion of the circum-Greenland reservoir age database is therefore not strictly limited to samples from surface waters but includes many samples from deeper sites on the shelf and even a few from the deep ocean (Fig. 2). The largest range of ΔR values is found in the surface ocean above approximately 150 m (Fig. 3). Below this, the spread is less pronounced, and, generally, the ΔR values are closer to zero as one goes down the water column (Fig. 3). When calibrating benthic dates from deeper sites in areas where information on deep-water reservoir age is available, one could therefore consider excluding extreme values obtained from surface ocean samples when making the choice of which reservoir correction to apply. Not all zones include results from deeper sites, however, and the decreasing ΔR variability with depth can also partially be attributed to the lower number of data points available for deeper sites (Fig. 3). The few measurements of samples from water depths beyond >1000 m are from sites in the Greenland Sea, Norwegian Sea, and Labrador Sea (Fig. 1). These are all at locations characterized by convection and formation of North Atlantic Deep Water and Labrador Sea Water (Broecker, 1991; Smethie et al., 2000), which is the likely explanation for the low ΔR values indicating deep waters younger than the average global surface ocean (Fig. 3). Zone 5 also includes multiple deep sites on the northwest Greenland shelf (Fig. 2) with water depths exceeding 500 m accompanied by relatively low ΔR values (Fig. 3). These younger waters at depth could be attributed to Atlantic Water sourced from the East Greenland Current but also possibly Labrador Sea waters, transported north into the West Greenland Current.
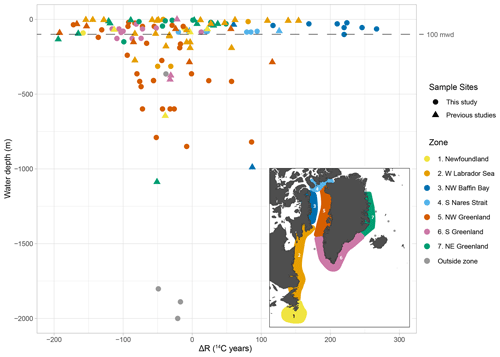
Figure 3Scatterplot of ΔR values versus water depth of individual sites, colored according to the geographic zone shown in the insert, as defined in Fig. 2. Water depth values for the new sites of this study were obtained from museum catalogues, while the water depth of previous studies was retrieved by finding the GEBCO water depth (GEBCO Bathymetric Compilation Group, 2022) for the coordinates listed in the marine reservoir database of Reimer and Reimer (2001). The horizontal and vertical axes are cropped at ΔR = 300 years and 2000 m water depth, respectively, to improve the readability of the graph. This resulted in two data points falling outside the plotted area, namely no. 61 (ΔR = −149 ± 27 14C years, 2750 m wd) and no. 15 (ΔR = 546 ± 25 14C years, 20 m wd); see also Table S1.
4.3 Sea ice
Sea ice acts as a physical barrier between the ocean and the atmosphere and prevents the uptake of atmospheric 14CO2 in surface waters, thereby increasing the local marine reservoir age. This link has been well-established and is one of the main reasons why the latest calibration curve Marine20 is described as not suitable for application in polar regions where sea-ice cover impacts the reservoir age (Heaton et al., 2020). Model experiments suggest a direct relationship between the average annual duration of sea-ice cover and the magnitude of the local reservoir age ΔR (Bard et al., 1994), and sea-ice variability in the past has been found to play an important role in ΔR fluctuations on millennial timescales (Butzin et al., 2017). Sea-ice conditions around Greenland and the adjacent seas cover the full range of year-round ice-free conditions in the south to near-perennial sea-ice cover offshore northeast Greenland, making this study area ideal for investigating the link between sea-ice concentration and ΔR. Although there is no direct clear linear relationship between the two variables based on the entire dataset, the highest ΔR values are typically found in areas with elevated sea-ice cover (Fig. 4). At sites with annual average sea-ice concentrations of less than 25 %, no ΔR values higher than 50 14C years are found, and where ΔR values exceed 160 14C years, the sea-ice concentrations are >75 % (Fig. 4). Within each of the individual geographic zones as defined in Fig. 2, several positive correlations appear (Fig. 4), but the only significant positive correlation between sea-ice cover and reservoir age is found in Zone 2. No relationship at all between the variables, or even a minor non-significant negative correlation, is found in Zone 1 around Newfoundland with predominantly ice-free conditions and Zone 4 around Nares Strait (Fig. 4). As discussed above, there are other factors influencing the reservoir age, and in this specific case, the region with the highest ΔR values (Zone 3, NW Baffin), is not only characterized by elevated sea-ice concentrations but also the influence of Arctic and Pacific waters and carbonate bedrock, all together contributing to the older waters.
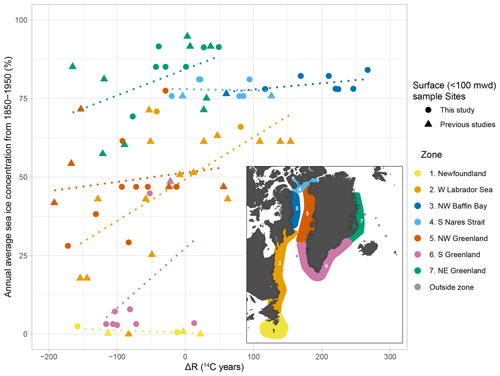
Figure 4Scatterplot of ΔR values of surface waters (<100 m wd) versus historical sea-ice concentrations of individual sites, colored according to the geographic zone shown in the insert, as defined in Fig. 2. The dotted lines represent linear trends for each of the zones, colored accordingly. The only significant correlation is found in Zone 2, in the western Labrador Sea. Sea ice for each site is the annual average value for the period from 1850–1950, derived from Walsh et al. (2017). One data point lies outside the plotted area (no. 15, ΔR = 546 ± 25 14C years, sea ice 76 %), since the horizontal axis is cropped at ΔR = 300 years to improve the readability of the graph.
4.4 Mollusk feeding habits
A potential obstacle in using marine mollusks as recorders of the 14C content of the surrounding ocean water is the variability in feeding habits of different species. Depending on the feeding habit, individual species may be taking up carbon from different pools. Suspension feeders, as the name implies, feed on organic matter that is suspended in the water column, while deposit feeders take up carbon from material that has settled on the sea floor. This is of great importance in areas with carbonate bedrock, where sediment pore water may contain old bicarbonate which would offset the age of deposit feeding mollusks. This phenomenon has also been called the “Portlandia effect”, named after the deposit-feeding mollusk Portlandia arctica which was found to be up to several thousand years older than its suspension-feeding counterparts in a study from the Canadian Arctic Archipelago (England et al., 2013). The circum-Greenland dataset analyzed here consists mostly of suspension feeders but also of numerous deposit feeders. The most common suspension feeders in this study are Astarte borealis, Hiatella arctica, and Mytilus edulis, while the most common deposit feeders are Macoma calcarea and Nuculana permula (Table S1). A direct comparison shows no consistent offset between the two groups, although the deposit feeders do show a tendency towards slightly higher ΔR values (Fig. 5). The highest value in the entire dataset is ΔR = 546 ± 25 years, measured in a specimen of Macoma calcarea from the northern coast of Bylot Island in Lancaster Sound (sample no. 15, Fig. S1). This anomalously high value, combined with the knowledge that it is retrieved from a deposit feeder in a region that is characterized by carbonate bedrock is thus very likely a result of the Portlandia effect, and the date should not be considered reliable. In conclusion, results from the deposit feeders are mostly compatible with those from suspension feeders (Fig. 5), but caution should be taken in areas with carbonate bedrock, where the deposit feeders should be avoided.
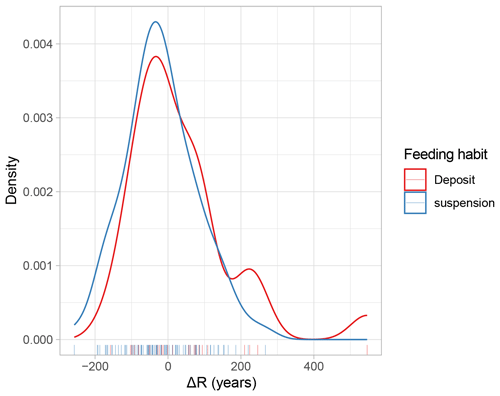
Figure 5Density plots of ΔR values colored by feeding habit of mollusks. Deposit feeders are plotted in red (n=27) and suspension feeders in blue (n=98). Information on mollusk feeding habits was obtained from the marine radiocarbon database (Reimer and Reimer, 2001), supplemented by information published in the World Register of Marine Species (WoRMS Editorial Board, 2023).
This study provides a significant expansion of the available data of the marine reservoir age around Greenland and the adjacent seas. All new data were obtained from radiocarbon measurements on mollusks (n=92) stored in museum collections in Stockholm and Copenhagen. The new data include many sites from deeper water depths, beyond the surface mixed layer which is traditionally used for the determination of the marine reservoir age. This expansion to deeper water depths, allows the inclusion of samples which are located further offshore and provides a broader representation of sites that are typically cored for paleoceanographic studies. When our new data are combined with results from previous studies in the area, the following conclusions can be drawn.
-
Museum sample storage. The exact age of samples from “dry” collections is possibly unknown, and thus, where possible, preferably only samples with soft tissue present, stored in “wet” collections, should be used for ΔR measurements. If dry samples are the only option, they should be carefully examined for any signs of age or reworking after death (O'Connor et al., 2010).
-
Water masses and ocean currents. The youngest waters are found in areas dominated by Atlantic Water, while the oldest are found in regions influenced by Arctic Ocean outflow and Pacific Water.
-
Water depth. The largest variance in ΔR is found in the surface waters. Further down the water column, the ΔR values become less variable and are closer to zero. In areas of deepwater formation, even sites beyond 1500 m water depth have ΔR values <0 14C years.
-
Sea ice. On a regional scale, our data suggest a clear link between sea-ice cover and the radiocarbon age of the underlying waters. The highest ΔR values are found in regions with high (< 75 %) annual sea-ice cover, while areas with almost no sea-ice cover have typically low (ΔR < 50 14C years) values.
-
Suspension vs. deposit feeders. In general, ΔR measurements on mollusks with either feeding habit are compatible. In regions with carbonate bedrock and the presence of old carbon, however, deposit feeders should be avoided, as they are found to overestimate the radiocarbon age of the waters.
For the calibration of radiocarbon dates, we provide regional averages as summarized in Table 1, but we encourage users to consult the full table of results provided in the Supplement. This allows one to choose a ΔR value based on prevailing water masses, water depth, distance to coast, mollusk species, and other factors, all together providing a value which is as representative of the study site as possible.
Despite this improved estimate of the regional reservoir age around Greenland, these values only remain valid for the pre-bomb, modern situation and most likely large parts of the current Holocene interglacial. Other studies have shown large temporal variability over millennial timescales linked to major changes in ocean circulation during the last glacial–interglacial transition (Skinner et al., 2019; Telesiński et al., 2021). Outside of the Holocene, our ΔR values provide a minimum bound for the calibration of radiocarbon ages, as also suggested in Heaton et al. (2023).
Although we provide a large expansion of spatial coverage around Greenland, large geographic areas remain unrepresented. No data at all are available for the northern coast of Greenland bordering the Arctic Ocean, above 76∘ N in east Greenland and 77∘ N in west Greenland in the Nares Strait.
All data presented in this study are available in the Supplement.
The supplement related to this article is available online at: https://doi.org/10.5194/gchron-5-451-2023-supplement.
CP: conceptualization, data curation, formal analysis, funding acquisition, investigation, project administration, supervision, visualization, writing – original draft preparation, writing – review and editing. KSÖ and RFM: formal analysis, investigation, writing – review and editing. HD: data curation, investigation, writing – review and editing. JO: conceptualization, formal analysis, supervision, writing – review and editing.
The contact author has declared that none of the authors has any competing interests.
Publisher’s note: Copernicus Publications remains neutral with regard to jurisdictional claims made in the text, published maps, institutional affiliations, or any other geographical representation in this paper. While Copernicus Publications makes every effort to include appropriate place names, the final responsibility lies with the authors.
Many thanks go to curators Mattias Forshage and particularly Anna Persson of the Swedish Museum of Natural History in Stockholm for providing access to the museum catalogues and the mollusk collections and for all the help with sampling. In Copenhagen, at the Zoological Museum (Natural History Museum of Denmark), we were welcomed by Tom Schiøtte, whose expertise on the Danish historical expeditions and mollusk collections was crucial for obtaining the samples needed for this study. Thanks go to Alexis Geels and Mériadec Le Pabic for help with sampling and photography of the catalogues and specimens during museum visits. Finally, many thanks go to Marie Kanstrup, technician at the Aarhus AMS Centre for the help with pre-treatment and analyses of radiocarbon measurements. This project was funded by the Independent Research Fund Denmark as project funding for CLAMS (Carbon Lag of Arctic Marginal Seas) to Christof Pearce (grant 8021-00148B).
This research has been supported by the Danmarks Frie Forskningsfond (grant no. 8021-00148B).
This paper was edited by Christine Hatté and reviewed by Paula Reimer, Matt O'Regan, and one anonymous referee.
Allaart, L., Schomacker, A., Larsen, N. K., Nørmark, E., Rydningen, T. A., Farnsworth, W. R., Retelle, M., Brynjólfsson, S., Forwick, M., and Kjellman, S. E.: Glacial history of the Åsgardfonna Ice Cap, NE Spitsbergen, since the last glaciation, Quaternary Sci. Rev., 251, 106717, https://doi.org/10.1016/j.quascirev.2020.106717, 2021.
Alves, E. Q., Macario, K., Ascough, P., and Bronk Ramsey, C.: The Worldwide Marine Radiocarbon Reservoir Effect: Definitions, Mechanisms, and Prospects, Rev. Geophys., 56, 278–305, https://doi.org/10.1002/2017RG000588, 2018.
Andresen, C. S., McCarthy, D. J., Dylmer, C. V., Seidenkrantz, M.-S., Kuijpers, A., and Lloyd, J. M.: Interaction between subsurface ocean waters and calving of the Jakobshavn Isbræ during the late Holocene, Holocene, 21, 211–224, https://doi.org/10.1177/0959683610378877, 2011.
Andresen, C. S., Hansen, M. J., Seidenkrantz, M.-S., Jennings, A. E., Knudsen, M. F., Nørgaard-Pedersen, N., Larsen, N. K., Kuijpers, A., and Pearce, C.: Mid- to late-Holocene oceanographic variability on the Southeast Greenland shelf, Holocene, 23, 167–178, https://doi.org/10.1177/0959683612460789, 2013.
Andrews, J. T. and Jennings, A. E.: Multidecadal to millennial marine climate oscillations across the Denmark Strait (∼ 66∘ N) over the last 2000 cal yr BP, Clim. Past, 10, 325–343, https://doi.org/10.5194/cp-10-325-2014, 2014.
Ascough, P., Cook, G., and Dugmore, A.: Methodological approaches to determining the marine radiocarbon reservoir effect, Prog. Phys. Geogr., 29, 532–547, https://doi.org/10.1191/0309133305pp461ra, 2005.
Audi, G., Bersillon, O., Blachot, J., and Wapstra, A. H.: The Nubase evaluation of nuclear and decay properties, Nucl. Phys. A, 729, 3–128, https://doi.org/10.1016/j.nuclphysa.2003.11.001, 2003.
Austin, W. E. N., Bard, E., Hunt, J. B., Kroon, D., and Peacock, J. D.: The 14C Age of the Icelandic Vedde Ash: Implications for Younger Dryas Marine Reservoir Age Corrections, Radiocarbon, 37, 53–62, https://doi.org/10.1017/S0033822200014788, 1995.
Bard, E., Arnold, M., Mangerud, J., Paterne, M., Labeyrie, L., Duprat, J., Mélières, M.-A., Sønstegaard, E., and Duplessy, J.-C.: The North Atlantic atmosphere-sea surface 14C gradient during the Younger Dryas climatic event, Earth Planet. Sc. Lett., 126, 275–287, https://doi.org/10.1016/0012-821X(94)90112-0, 1994.
Bevington, P. R. and Robinson, D. K.: Data Reduction and Error Analysis for the Physical Sciences, 3rd edn., McGraw-Hill, 320 pp., ISBN 978-0071199261, 2002.
Broecker, W.: The Great Ocean Conveyor, Oceanography, 4, 79–89, https://doi.org/10.5670/oceanog.1991.07, 1991.
Broecker, W. S., Gerard, R., Ewing, M., and Heezen, B. C.: Natural radiocarbon in the Atlantic Ocean, J. Geophys. Res., 65, 2903–2931, https://doi.org/10.1029/JZ065i009p02903, 1960.
Brouard, E., Roy, M., Godbout, P.-M., and Veillette, J. J.: A framework for the timing of the final meltwater outbursts from glacial Lake Agassiz-Ojibway, Quaternary Sci. Rev., 274, 107269, https://doi.org/10.1016/j.quascirev.2021.107269, 2021.
Butzin, M., Köhler, P., and Lohmann, G.: Marine radiocarbon reservoir age simulations for the past 50,000 years, Geophys. Res. Lett., 44, 8473–8480, https://doi.org/10.1002/2017GL074688, 2017.
Coulthard, R. D., Furze, M. F. A., Pieńkowski, A. J., Chantel Nixon, F., and England, J. H.: New marine ΔR values for Arctic Canada, Quat. Geochronol., 5, 419–434, https://doi.org/10.1016/j.quageo.2010.03.002, 2010.
Davies, J., Mathiasen, A. M., Kristiansen, K., Hansen, K. E., Wacker, L., Alstrup, A. K. O., Munk, O. L., Pearce, C., and Seidenkrantz, M.-S.: Linkages between ocean circulation and the Northeast Greenland Ice Stream in the Early Holocene, Quaternary Sci. Rev., 286, 107530, https://doi.org/10.1016/j.quascirev.2022.107530, 2022.
Devendra, D., Łącka, M., Telesiński, M. M., Rasmussen, T. L., Sztybor, K., and Zajączkowski, M.: Paleoceanography of the Northwestern Greenland Sea and Return Atlantic Current evolution, 35–4 kyr BP, Global Planet. Change, 217, 103947, https://doi.org/10.1016/j.gloplacha.2022.103947, 2022.
El bani Altuna, N., Ezat, M. M., Greaves, M., and Rasmussen, T. L.: Millennial-Scale Changes in Bottom Water Temperature and Water Mass Exchange Through the Fram Strait 79∘ N, 63–13 ka, Paleoceanogr. Paleocl., 36, e2020PA004061, https://doi.org/10.1029/2020PA004061, 2021.
England, J., Dyke, A. S., Coulthard, R. D., Mcneely, R., and Aitken, A.: The exaggerated radiocarbon age of deposit-feeding molluscs in calcareous environments, Boreas, 42, 362–373, https://doi.org/10.1111/j.1502-3885.2012.00256.x, 2013.
Erbs-Hansen, D. R., Knudsen, K. L., Olsen, J., Lykke-Andersen, H., Underbjerg, J. A., and Sha, L.: Paleoceanographical development off Sisimiut, West Greenland, during the mid- and late Holocene: A multiproxy study, Mar. Micropaleontol., 102, 79–97, https://doi.org/10.1016/j.marmicro.2013.06.003, 2013.
Forman, S. L. and Polyak, L.: Radiocarbon content of pre-bomb marine mollusks and variations in the 14C Reservoir age for coastal areas of the Barents and Kara Seas, Russia, Geophys. Res. Lett., 24, 885–888, https://doi.org/10.1029/97GL00761, 1997.
GEBCO Bathymetric Compilation Group: GEBCO_2022 Grid, British Oceanographic Data Centre, National Oceanography Centre, NERC, UK, https://doi.org/10.5285/e0f0bb80-ab44-2739-e053-6c86abc0289c, 2022.
Glueder, A., Mix, A. C., Milne, G. A., Reilly, B. T., Clark, J., Jakobsson, M., Mayer, L., Fallon, S. J., Southon, J., Padman, J., Ross, A., Cronin, T., and McKay, J. L.: Calibrated relative sea levels constrain isostatic adjustment and ice history in northwest Greenland, Quaternary Sci. Rev., 293, 107700, https://doi.org/10.1016/j.quascirev.2022.107700, 2022.
Hansen, K. E., Giraudeau, J., Wacker, L., Pearce, C., and Seidenkrantz, M.-S.: Reconstruction of Holocene oceanographic conditions in eastern Baffin Bay, Clim. Past, 16, 1075–1095, https://doi.org/10.5194/cp-16-1075-2020, 2020.
Hansen, K. E., Lorenzen, J., Davies, J., Wacker, L., Pearce, C., and Seidenkrantz, M.-S.: Deglacial to Mid Holocene environmental conditions on the northeastern Greenland shelf, western Fram Strait, Quaternary Sci. Rev., 293, 107704, https://doi.org/10.1016/j.quascirev.2022.107704, 2022.
Heaton, T. J., Köhler, P., Butzin, M., Bard, E., Reimer, R. W., Austin, W. E. N., Ramsey, C. B., Grootes, P. M., Hughen, K. A., Kromer, B., Reimer, P. J., Adkins, J., Burke, A., Cook, M. S., Olsen, J., and Skinner, L. C.: Marine20—The Marine Radiocarbon Age Calibration Curve (0–55,000 cal BP), Radiocarbon, 62, 779–820, https://doi.org/10.1017/RDC.2020.68, 2020.
Heaton, T. J., Bard, E., Bronk Ramsey, C., Butzin, M., Köhler, P., Muscheler, R., Reimer, P. J., and Wacker, L.: Radiocarbon: A key tracer for studying Earth's dynamo, climate system, carbon cycle, and Sun, Science, 374, eabd7096, https://doi.org/10.1126/science.abd7096, 2021.
Heaton, T. J., Bard, E., Ramsey, C. B., Butzin, M., Hatté, C., Hughen, K. A., Köhler, P., and Reimer, P. J.: A RESPONSE TO COMMUNITY QUESTIONS ON THE MARINE20 RADIOCARBON AGE CALIBRATION CURVE: MARINE RESERVOIR AGES AND THE CALIBRATION OF 14C SAMPLES FROM THE OCEANS, Radiocarbon, 65, 247–273, https://doi.org/10.1017/RDC.2022.66, 2023.
Hesshaimer, V., Heimann, M., and Levin, I.: Radiocarbon evidence for a smaller oceanic carbon dioxide sink than previously believed, Nature, 370, 201–203, https://doi.org/10.1038/370201a0, 1994.
Hjort, C.: A sea correction for East Greenland, Geologiska Fören. Stock. F., 95, 132–134, https://doi.org/10.1080/11035897309455434, 1973.
Hogg, A. G., Heaton, T. J., Hua, Q., Palmer, J. G., Turney, C. S., Southon, J., Bayliss, A., Blackwell, P. G., Boswijk, G., Ramsey, C. B., Pearson, C., Petchey, F., Reimer, P., Reimer, R., and Wacker, L.: SHCal20 Southern Hemisphere Calibration, 0–55,000 Years cal BP, Radiocarbon, 62, 759–778, https://doi.org/10.1017/RDC.2020.59, 2020.
Hua, Q., Webb, G. E., Zhao, J., Nothdurft, L. D., Lybolt, M., Price, G. J., and Opdyke, B. N.: Large variations in the Holocene marine radiocarbon reservoir effect reflect ocean circulation and climatic changes, Earth Planet. Sc. Lett., 422, 33–44, https://doi.org/10.1016/j.epsl.2015.03.049, 2015.
Jackson, R., Andreasen, N., Oksman, M., Andersen, T. J., Pearce, C., Seidenkrantz, M.-S., and Ribeiro, S.: Marine conditions and development of the Sirius Water polynya on the North-East Greenland shelf during the Younger Dryas-Holocene, Quaternary Sci. Rev., 291, 107647, https://doi.org/10.1016/j.quascirev.2022.107647, 2022.
Jennings, A., Andrews, J., and Wilson, L.: Holocene environmental evolution of the SE Greenland Shelf North and South of the Denmark Strait: Irminger and East Greenland current interactions, Quaternary Sci. Rev., 30, 980–998, https://doi.org/10.1016/j.quascirev.2011.01.016, 2011.
Jensen, K. G., Kuijpers, A., Koc, N., and Heinemeier, J.: Diatom evidence of hydrographic changes and ice conditions in Igaliku Fjord, South Greenland, during the past 1500 years, Holocene, 14, 152–164, https://doi.org/10.1191/0959683604hl698rp, 2004.
Jones, E. P., Swift, J. H., Anderson, L. G., Lipizer, M., Civitarese, G., Falkner, K. K., Kattner, G., and McLaughlin, F.: Tracing Pacific water in the North Atlantic Ocean, J. Geophys. Res.-Oceans, 108, 3116, https://doi.org/10.1029/2001JC001141, 2003.
Kimoto, K.: Planktic Foraminifera, in: Marine Protists: Diversity and Dynamics, edited by: Ohtsuka, S., Suzaki, T., Horiguchi, T., Suzuki, N., and Not, F., Springer Japan, Tokyo, 129–178, https://doi.org/10.1007/978-4-431-55130-0_7, 2015.
Knudsen, K. L., Stabell, B., Seidenkrantz, M.-S., Eiríksson, J., and Blake, W.: Deglacial and Holocene conditions in northernmost Baffin Bay: sediments, foraminifera, diatoms and stable isotopes, Boreas, 37, 346–376, https://doi.org/10.1111/j.1502-3885.2008.00035.x, 2008.
Krawczyk, D. W., Witkowski, A., Moros, M., Lloyd, J. M., Høyer, J. L., Miettinen, A., and Kuijpers, A.: Quantitative reconstruction of Holocene sea ice and sea surface temperature off West Greenland from the first regional diatom data set: Holocene Paleoceanography in West Greenland, Paleoceanography, 32, 18–40, https://doi.org/10.1002/2016PA003003, 2017.
Lassen, S. J., Kuijpers, A., Kunzendorf, H., Hoffmann-Wieck, G., Mikkelsen, N., and Konradi, P.: Late-Holocene Atlantic bottom-water variability in Igaliku Fjord, South Greenland, reconstructed from foraminifera faunas, Holocene, 14, 165–171, https://doi.org/10.1191/0959683604hl699rp, 2004.
Levac, E., Vernal, A. D., and Blake Jr., W.: Sea-surface conditions in northernmost Baffin Bay during the Holocene: palynological evidence, J. Quaternary Sci., 16, 353–363, https://doi.org/10.1002/jqs.614, 2001.
Li, D., Sha, L., Li, J., Jiang, H., Liu, Y., and Wu, Y.: Summer Sea-Surface Temperatures and Climatic Events in Vaigat Strait, West Greenland, during the Last 5000 Years, Sustainability, 9, 704, https://doi.org/10.3390/su9050704, 2017.
Liljequist, G. H.: High latitudes: A history of Swedish Polar travels and research, Swedish Polar Research Secretariat in collaboration with Streiffert, Stockholm, 607 pp., ISBN 91-7886-102-0, 1993.
Lloyd, J., Moros, M., Perner, K., Telford, R. J., Kuijpers, A., Jansen, E., and McCarthy, D.: A 100 yr record of ocean temperature control on the stability of Jakobshavn Isbrae, West Greenland, Geology, 39, 867–870, https://doi.org/10.1130/G32076.1, 2011.
Lloyd, J. M.: Late Holocene environmental change in Disko Bugt, west Greenland: interaction between climate, ocean circulation and Jakobshavn Isbrae, Boreas, 35, 35–49, https://doi.org/10.1111/j.1502-3885.2006.tb01111.x, 2006.
Lougheed, B. C., Filipsson, H. L., and Snowball, I.: Large spatial variations in coastal 14C reservoir age – a case study from the Baltic Sea, Clim. Past, 9, 1015–1028, https://doi.org/10.5194/cp-9-1015-2013, 2013.
Mangerud, J.: Radiocarbon dating of marine shells, including a discussion of apparent age of Recent shells from Norway, Boreas, 1, 143–172, https://doi.org/10.1111/j.1502-3885.1972.tb00147.x, 1972.
Mangerud, J., Bondevik, S., Gulliksen, S., Karin Hufthammer, A., and Høisæter, T.: Marine 14C reservoir ages for 19th century whales and molluscs from the North Atlantic, Quaternary Sci. Rev., 25, 3228–3245, https://doi.org/10.1016/j.quascirev.2006.03.010, 2006.
Matsumoto, K.: Radiocarbon-based circulation age of the world oceans, J. Geophys. Res.-Oceans, 112, C09004, https://doi.org/10.1029/2007JC004095, 2007.
McNeely, R., Dyke, A. S., and Southon, J. R.: Canadian marine reservoir ages, preliminary data assessment, Geological Survey of Canada, Open File 5049, 2006, 3 pp., 1 CD-ROM, https://doi.org/10.4095/221564, 2006.
Millan, R., Rignot, E., Mouginot, J., Wood, M., Bjørk, A. A., and Morlighem, M.: Vulnerability of Southeast Greenland Glaciers to Warm Atlantic Water From Operation IceBridge and Ocean Melting Greenland Data, Geophys. Res. Lett., 45, 2688–2696, https://doi.org/10.1002/2017GL076561, 2018.
Møller, H. S., Jensen, K. G., Kuijpers, A., Aagaard-Sørensen, S., Seidenkrantz, M.-S., Prins, M., Endler, R., and Mikkelsen, N.: Late-Holocene environment and climatic changes in Ameralik Fjord, southwest Greenland: evidence from the sedimentary record, Holocene, 16, 685–695, https://doi.org/10.1191/0959683606hl963rp, 2006.
Moros, M., Jensen, K. G., and Kuijpers, A.: Mid-to late-Holocene hydrological and climatic variability in Disko Bugt, central West Greenland, Holocene, 16, 357–367, https://doi.org/10.1191/0959683606hl933rp, 2006.
Moros, M., Lloyd, J. M., Perner, K., Krawczyk, D., Blanz, T., de Vernal, A., Ouellet-Bernier, M.-M., Kuijpers, A., Jennings, A. E., Witkowski, A., Schneider, R., and Jansen, E.: Surface and sub-surface multi-proxy reconstruction of middle to late Holocene palaeoceanographic changes in Disko Bugt, West Greenland, Quaternary Sci. Rev., 132, 146–160, https://doi.org/10.1016/j.quascirev.2015.11.017, 2016.
Nathorst, A. G.: Två somrar I Norra Ishafvet: Kung Karls Land, Spetsbergens kringsegling, spanande efter Andrée, i nordöstra Grönland, Beijers Bokförlagsaktiebolag, Stockholm, 1900.
Nørgaard-Pedersen, N. and Mikkelsen, N.: 8000 year marine record of climate variability and fjord dynamics from Southern Greenland, Mar. Geol., 264, 177–189, https://doi.org/10.1016/j.margeo.2009.05.004, 2009.
O'Connor, S., Ulm, S., Fallon, S. J., Barham, A., and Loch, I.: Pre-Bomb Marine Reservoir Variability in the Kimberley Region, Western Australia, Radiocarbon, 52, 1158–1165, https://doi.org/10.1017/S0033822200046233, 2010.
Olsen, I. L., Laberg, J. S., Forwick, M., Rydningen, T. A., and Husum, K.: Late Weichselian and Holocene behavior of the Greenland Ice Sheet in the Kejser Franz Josef Fjord system, NE Greenland, Quaternary Sci. Rev., 284, 107504, https://doi.org/10.1016/j.quascirev.2022.107504, 2022.
Olsen, J., Rasmussen, T. L., and Reimer, P. J.: North Atlantic marine radiocarbon reservoir ages through Heinrich event H4: a new method for marine age model construction, Geol. Soc. Lond. Spec. Publ., 398, SP398.2, https://doi.org/10.1144/SP398.2, 2014.
Olsen, J., Tikhomirov, D., Grosen, C., Heinemeier, J., and Klein, M.: Radiocarbon Analysis on the New AARAMS 1MV Tandetron, Radiocarbon, 59, 905–913, https://doi.org/10.1017/RDC.2016.85, 2017.
Olsson, I. U.: Content of C-14 in marine mammals from northern Europe, Radiocarbon, 22, 662–675, https://doi.org/10.1017/S0033822200010031, 1980.
Ouellet-Bernier, M.-M., Vernal, A. de, Hillaire-Marcel, C., and Moros, M.: Paleoceanographic changes in the Disko Bugt area, West Greenland, during the Holocene, Holocene, 24, 1573–1583, https://doi.org/10.1177/0959683614544060, 2014.
Pados-Dibattista, T., Pearce, C., Detlef, H., Bendtsen, J., and Seidenkrantz, M.-S.: Holocene palaeoceanography of the Northeast Greenland shelf, Clim. Past, 18, 103–127, https://doi.org/10.5194/cp-18-103-2022, 2022.
Pearce, C., Varhelyi, A., Wastegård, S., Muschitiello, F., Barrientos, N., O'Regan, M., Cronin, T. M., Gemery, L., Semiletov, I., Backman, J., and Jakobsson, M.: The 3.6 ka Aniakchak tephra in the Arctic Ocean: a constraint on the Holocene radiocarbon reservoir age in the Chukchi Sea, Climate of the Past, 13, 303–316, https://doi.org/10.5194/cp-13-303-2017, 2017.
Peral, M., Austin, W. E. N., and Noormets, R.: Identification of Atlantic water inflow on the north Svalbard shelf during the Holocene, J. Quaternary Sci., 37, 86–99, https://doi.org/10.1002/jqs.3374, 2022.
Perner, K., Moros, M., Snowball, I., Lloyd, J. M., Kuijpers, A., and Richter, T.: Establishment of modern circulation pattern at c. 6000 cal a BP in Disko Bugt, central West Greenland: opening of the Vaigat Strait, J. Quaternary Sci., 28, 480–489, https://doi.org/10.1002/jqs.2638, 2013.
Pieńkowski, A. J., Coulthard, R. D., and Furze, M. F. A.: Revised marine reservoir offset (ΔR) values for molluscs and marine mammals from Arctic North America, Boreas, 52, 145–167, https://doi.org/10.1111/bor.12606, 2022.
Reimer, P. J. and Reimer, R. W.: A marine reservoir correction database and on-line interface, Radiocarbon, 43, 461–463, https://doi.org/10.1017/S0033822200038339, 2001.
Reimer, P. J., Bard, E., Bayliss, A., Beck, J. W., Blackwell, P. G., Bronk Ramsey, C., Grootes, P. M., Guilderson, T. P., Haflidason, H., Hajdas, I., Hatté, C., Heaton, T. J., Hoffmann, D. L., Hogg, A. G., Hughen, K. A., Kaiser, K. F., Kromer, B., Manning, S. W., Niu, M., Reimer, R. W., Richards, D. A., Scott, E. M., Southon, J. R., Staff, R. A., Turney, C. S. M., and van der Plicht, J.: IntCal13 and Marine13 Radiocarbon Age Calibration Curves 0–50,000 Years cal BP, Radiocarbon, 55, 1869–1887, https://doi.org/10.2458/azu_js_rc.55.16947, 2013.
Reimer, P. J., Austin, W. E. N., Bard, E., Bayliss, A., Blackwell, P. G., Ramsey, C. B., Butzin, M., Cheng, H., Edwards, R. L., Friedrich, M., Grootes, P. M., Guilderson, T. P., Hajdas, I., Heaton, T. J., Hogg, A. G., Hughen, K. A., Kromer, B., Manning, S. W., Muscheler, R., Palmer, J. G., Pearson, C., Plicht, J. van der, Reimer, R. W., Richards, D. A., Scott, E. M., Southon, J. R., Turney, C. S. M., Wacker, L., Adolphi, F., Büntgen, U., Capano, M., Fahrni, S. M., Fogtmann-Schulz, A., Friedrich, R., Köhler, P., Kudsk, S., Miyake, F., Olsen, J., Reinig, F., Sakamoto, M., Sookdeo, A., and Talamo, S.: The IntCal20 Northern Hemisphere Radiocarbon Age Calibration Curve (0–55 cal kBP), Radiocarbon, 62, 725–757, https://doi.org/10.1017/RDC.2020.41, 2020.
Reimer, R. W. and Reimer, P. J.: An Online Application for ΔR Calculation, Radiocarbon, 59, 1623–1627, https://doi.org/10.1017/RDC.2016.117, 2017.
Riis-Carstensen, E.: Nogle Bemærkninger vedrørende Godthaab ekspeditionen 1928, Geografisk Tidsskrift, 1929.
Schaffer, J., Kanzow, T., von Appen, W.-J., von Albedyll, L., Arndt, J. E., and Roberts, D. H.: Bathymetry constrains ocean heat supply to Greenland's largest glacier tongue, Nat. Geosci., 13, 227–231, https://doi.org/10.1038/s41561-019-0529-x, 2020.
Seidenkrantz, M. S., Roncaglia, L., Fischel, A., Heilmann-Clausen, C., Kuijpers, A., and Moros, M.: Variable North Atlantic climate seesaw patterns documented by a late Holocene marine record from Disko Bugt, West Greenland, Mar. Micropaleontol., 68, 66–83, https://doi.org/10.1016/j.marmicro.2008.01.006, 2008.
Selin, N. I.: The growth and life span of bivalve mollusks at the northeastern coast of Sakhalin Island, Russ. J. Mar. Biol., 36, 258–269, https://doi.org/10.1134/S1063074010040048, 2010.
Sha, L., Knudsen, K. L., Eiríksson, J., Björck, S., Jiang, H., Yang, X., Yu, X., and Li, D.: Diatom-reconstructed summer sea-surface temperatures and climatic events off North Iceland during the last deglaciation and Holocene, Palaeogeogr. Palaeocl., 602, 111154, https://doi.org/10.1016/j.palaeo.2022.111154, 2022.
Skinner, L. C., Muschitiello, F., and Scrivner, A. E.: Marine Reservoir Age Variability Over the Last Deglaciation: Implications for Marine CarbonCycling and Prospects for Regional Radiocarbon Calibrations, Paleoceanogr. Paleocl., 34, 1807–1815, https://doi.org/10.1029/2019PA003667, 2019.
Smethie Jr., W. M., Fine, R. A., Putzka, A., and Jones, E. P.: Tracing the flow of North Atlantic Deep Water using chlorofluorocarbons, J. Geophys. Res.-Oceans, 105, 14297–14323, https://doi.org/10.1029/1999JC900274, 2000.
Stevenard, N., Montero-Serrano, J.-C., Eynaud, F., St-Onge, G., Zaragosi, S., and Copland, L.: Lateglacial and Holocene sedimentary dynamics in northwestern Baffin Bay as recorded in sediment cores from Cape Norton Shaw Inlet (Nunavut, Canada), Boreas, 51, 532–552, https://doi.org/10.1111/bor.12575, 2022.
Stuiver, M. and Braziunas, T. F.: Sun, ocean, climate and atmospheric 14CO2: an evaluation of causal and spectral relationships, Holocene, 3, 289–305, https://doi.org/10.1177/095968369300300401, 1993.
Stuiver, M. and Polach, H. A.: Discussion: reporting of 14C data, Radiocarbon, 19, 355–363, 1977.
Stuiver, M., Pearson, G. W., and Braziunas, T.: Radiocarbon Age Calibration of Marine Samples Back to 9000 Cal Yr BP, Radiocarbon, 28, 980–1021, https://doi.org/10.1017/S0033822200060264, 1986.
Sutherland, D. A., Straneo, F., Stenson, G. B., Davidson, F. J. M., Hammill, M. O., and Rosing-Asvid, A.: Atlantic water variability on the SE Greenland continental shelf and its relationship to SST and bathymetry, J. Geophys. Res.-Oceans, 118, 847–855, https://doi.org/10.1029/2012JC008354, 2013.
Tauber, H. and Funder, S.: C14 content of recent molluscs from Scoresby Sund, central East Greenland, Geological Survey of Greenland, 75, 95–99, 1975.
Telesiński, M. M., Ezat, M. M., Muschitiello, F., Bauch, H. A., and Spielhagen, R. F.: Ventilation History of the Nordic Seas Deduced From Pelagic-Benthic Radiocarbon Age Offsets, Geochem. Geophy. Geosy., 22, e2020GC009132, https://doi.org/10.1029/2020GC009132, 2021.
Walsh, J. E., Fetterer, F., Scott Stewart, J., and Chapman, W. L.: A database for depicting Arctic sea ice variations back to 1850, Geogr. Rev., 107, 89–107, https://doi.org/10.1111/j.1931-0846.2016.12195.x, 2017.
Wanamaker, A. D., Heinemeier, J., Scourse, J. D., Richardson, C. A., Butler, P. G., Eiríksson, J., and Knudsen, K. L.: Very Long-Lived Mollusks Confirm 17th Century AD Tephra-Based Radiocarbon Reservoir Ages for North Icelandic Shelf Waters, Radiocarbon, 50, 399–412, https://doi.org/10.1017/S0033822200053510, 2008.
West, G., Nilsson, A., Geels, A., Jakobsson, M., Moros, M., Muschitiello, F., Pearce, C., Snowball, I., and O'Regan, M.: Late Holocene Paleomagnetic Secular Variation in the Chukchi Sea, Arctic Ocean, Geochem. Geophy. Geosy., 23, e2021GC010187, https://doi.org/10.1029/2021GC010187, 2022.
WoRMS Editorial Board: World Register of Marine Species, VLIZ, https://www.marinespecies.org (last access: 4 April 2023), 2023.