the Creative Commons Attribution 4.0 License.
the Creative Commons Attribution 4.0 License.
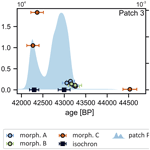
230Th ∕ U isochron dating of cryogenic cave carbonates
Paul Töchterle
Simon D. Steidle
R. Lawrence Edwards
Yuri Dublyansky
Christoph Spötl
Xianglei Li
John Gunn
Gina E. Moseley
Cryogenic cave carbonates (CCCs) are a type of speleothem, typically dated with disequilibrium methods, that provide evidence of palaeo-permafrost conditions. In the field, CCCs occur as distinct patches of millimetre- to centimetre-sized loose crystals and crystal aggregates on the floors of cave chambers, so they lack a framework that would allow ages to be validated by stratigraphic order. Correction factors for the initial 230Th (230Th0) are often based on the bulk-earth-derived initial activity ratio (), which is a well-established approach when 230Th0 is moderately low. For samples with elevated levels of 230Th0, however, accuracy can be improved by constraining independently. Here, we combine detailed morphological observations from three CCC patches found in Water Icicle Close Cavern in the Peak District (UK) with analyses. We find that individual CCC crystals show a range of morphologies that arise from non-crystallographic branching in response to the chemical evolution of the freezing solution. Results of dating indicate that samples with a large surface area relative to the sample volume are systematically more affected by contamination with 230Th0. By fitting isochrons to these results, we test whether the CCCs in a patch formed during the same freezing event, and demonstrate that can deviate substantially from the bulk-earth-derived value and can also vary between the different CCC patches. Where CCCs display elevated 230Th0, isochrons are a useful tool to constrain and obtain ages with improved accuracy. Detritus absorbed to the crystal surface is shown to be the most likely source of 230Th0. Our results suggest that some previously published CCC ages may merit re-assessment, and we provide suggestions on how to approach future dating efforts.
- Article
(4199 KB) - Full-text XML
-
Supplement
(1031 KB) - BibTeX
- EndNote
Cryogenic cave carbonates (CCCs) are speleothems that typically occur as an accumulation of loose crystals on the floors of cave chambers. Despite being described in earlier literature (Skřivánek, 1954), it was not until the early 2000s that their value as palaeoclimate archives became apparent (Žák et al., 2004). The widely accepted model of CCC formation involves precipitation in slowly freezing pools of water on the tops of cave ice bodies. This particular setting requires pre-existing sizeable bodies of cave ice with cave air temperatures between approximately −1 and 0 ∘C (i.e. the “CCC window”, Spötl et al., 2021; Koltai et al., 2021), indicating that the cave was surrounded by permafrost at the time of CCC formation. A study of Winter Wonderland Cave, USA (Munroe et al., 2021), validated this genetic model by observing very recent formation of CCCs in the exact setting proposed by Žák et al. (2004).
As with common speleothems (e.g. stalagmites and flowstones), the formation age of CCCs is determined by disequilibrium dating. The principle of this method relies on the large solubility difference between U and Th in typical karst water (Gascoyne, 1992). An age precision as good as 2 ‰ is routinely achieved using the latest analytical protocol (Cheng et al., 2013). However, the accuracy of dating results can be compromised if substantial amounts of Th incorporated at the time of speleothem formation (230Th0) are not adequately corrected for (Dorale et al., 2004). In practice, 230Th0 is identified and corrected by measuring the chemically equivalent 232Th, a long-lived isotope that is not part of the 238U decay chain. This measurement is hereafter reported as the modern activity ratio , where the subscript t signifies time after formation and the parentheses indicate an activity ratio (as opposed to an atomic ratio). Subsequently, 230Th0 is subtracted by applying the initial activity ratio (()0).
However, in nature, () varies by orders of magnitude (values from 0.8 to 73 have been reported in Scott, 1968; Moore and Sackett, 1964; Moore, 1981; Hubert et al., 2006; and Hirose et al., 2012). The large spread of () is attributed to varying contributions of multiple reservoirs of Th in the respective aqueous environment: typically a mix of detrital particles (such as clay minerals, alumosilicates, or iron (oxyhydr)oxides), colloidal phases, organics and carbonate complexes (Richards and Dorale, 2003). Consequently, needs to be accurately constrained if a sample's 230Th0 is high enough to make the age equation sensitive to the correction term. There are four approaches to deriving : (1) by using the bulk-earth composition (e.g. Wedepohl, 1995); (2) by deriving it from stratigraphic constraints (e.g. Cheng et al., 2000; Hellstrom, 2006); (3) by using modern values measured at the field site, for example in groundwater; (4) by calculating values derived from isochrons (e.g. Ludwig and Titterington, 1994; Lin et al., 1998; Henderson and Slowey, 2000; Cobb et al., 2003; Moseley et al., 2015).
Since the early 2000s, more than 20 studies have published ages of CCCs, commonly from multiple samples, and interpreted them with regard to regional permafrost conditions (e.g. Richter and Riechelmann, 2008; Žák et al., 2009; Richter et al., 2010; Žák et al., 2012; Orvošová et al., 2014; Spötl and Cheng, 2014; Chaykovskiy et al., 2014; Richter et al., 2018; Koltai et al., 2021; Spötl et al., 2021). However, reported ages from a given patch of CCCs have rarely been synchronous and have sometimes spanned thousands of years and different climatic events (Luetscher et al., 2013; Dublyansky et al., 2018). This spread of ages has led investigators to conclude that in those cases, CCCs must have formed during multiple freezing events. Since CCCs typically occur as accumulations of crystals with no internal stratigraphy, it is generally not possible to constrain by stratigraphic order, validate individual ages, or detect potential outliers and/or erroneous measurements (i.e. “age reversals”); hence, in almost all cases, 230Th0 was corrected by assuming a bulk-earth composition for .
In this study, we apply the isochron dating technique to CCCs in order to constrain empirically. We present a comprehensive dataset of three CCC patches from Water Icicle Close Cavern comprising 26 ages, carbonate stable isotope data, and detailed morphological observations. We assess whether the isochron approach (i) can reliably identify multiple formation events within the achievable dating precision (which may or may not be represented in a given CCC patch) and (ii) can be used to precisely correct for 230Th0 in samples with substantial amounts of contamination.
The samples used in this study were collected from Water Icicle Close Cavern (WICC), a limestone dissolution cave located in the Peak District (United Kingdom). The cave entrance (53.1781∘ N, 1.7605∘ W) is situated 338 m above sea level and is surrounded by a low-gradient upland topography that has been incised by valleys, most of which are dry. The bedrock geology comprises a Lower Carboniferous limestone sequence several hundred metres thick. In total, the cave comprises over 1000 m of explored passages that formed along a horizontal level 30–40 m below the surface. Overall, 11 patches of CCC were documented in the cave, three of which were sampled in the course of this study. Additional information and a cave map are included in the Supplement.
The climate of the region is temperate, with a mean annual air temperature of 8.9 ∘C and 1160 mm of annual precipitation (UK METoffice, 2020). Previous research reported evidence of a former presence of extensive ice in the cave, including speleothem damage, ice attachments and solifluction deposits (Gunn et al., 2020). Dating of several broken and re-sealed speleothems points towards at least one period of cave glaciation between 87 000 and 83 000 years ago that can be related to the presence of permafrost. The area around Water Icicle Close Cavern was not glaciated during the Last Glacial Maximum, but the British–Irish Ice Sheet terminated only around 30 km northwest of the cave at its maximum extent (Clark et al., 2012).
3.1 Sampling strategy
Three patches of CCCs were sampled according to different strategies depending on the respective grain size of the occurrence. If the mean crystal size was larger than approximately 5 mm, several individual crystals were selected while trying to obtain a representative subsample of all morphological varieties of the patch. For smaller grain sizes, a subsample was simply scooped up with a knife. With all sampling, damage to the cave was minimised such that the overall appearance of the CCC patches was kept intact.
3.2 Carbonate stable isotope analysis
While CCCs can be identified by their characteristic crystal morphology and field occurrence, the diagnostic property separating them from common speleothems is their stable isotope composition (Žák et al., 2018). Several morphological varieties of each sampled CCC patch were selected for stable isotope analysis. If grain size permitted, aliquots were taken from the same specimen that was also used for petrographic analysis.
Samples were cleaned in an ultrasonic bath of deionised water before drilling between 0.10 and 0.80 mg of carbonate powder from random points on the surface with a carbide-burr-tipped dental drill. The stable isotope composition of these carbonate powders was then determined using a Thermo Scientific Delta V Plus isotope ratio mass spectrometer coupled to a GasBench II (Spötl and Vennemann, 2003). An analytical uncertainty (1σ) of ±0.06 ‰ for δ13C and ±0.08 ‰ for δ18O applies to all stable isotope data (Spötl, 2011), and values are reported relative to the Vienna PeeDee Belemnite (VPDB) standard.
3.3 230ThU analysis
As with the stable isotope analyses, smaller crystals were rinsed with deionised water for several minutes in an ultrasonic cleaner. Visible surficial detritus was removed with a toothbrush. Samples were then processed as a whole. For two large samples from patch 3 (PT25 and PT26, see Table 1), it was possible to cut them in half using a diamond-coated wire saw, polish them, and drill ca. 30 mg of powder form the inner part of the sample with a burr-tipped dental drill in a laminar-flow hood.
Table 1Results of analyses of CCC from Water Icicle Close Cavern. Uncertainties are reported at the 2σ level.
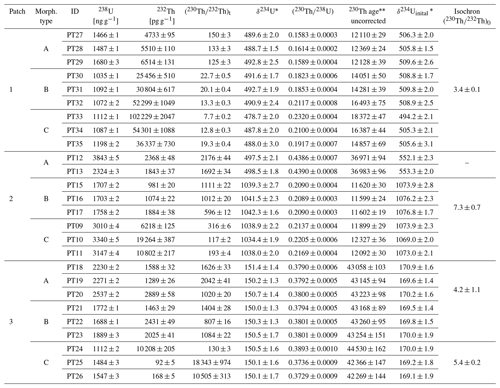
* δ234U .
Years BP.
Chemical preparation and mass spectrometry were performed at the Trace Metal Isotope Geochemistry Laboratory at the University of Minnesota. Elemental extraction of U and Th was performed according to the procedures described in Edwards et al. (1987). The resulting solutions were measured on a Thermo Fisher Neptune Plus multi-collector inductively coupled plasma mass spectrometer in peak-jumping mode on a secondary electron multiplier (Shen et al., 2012).
Ages were calculated according to Edwards et al. (1987) with decay constants of (Jaffey et al., 1971) and for U and for Th (Cheng et al., 2013). Measurement uncertainties are given at the 2σ level and ages are reported as years before 1950 (BP).
Each uncorrected age was iteratively corrected for 230Th0 based on a range of values, starting from a bulk-earth-derived value of 0.82±0.41, representing secular equilibrium with a () value of 3.8 (Wedepohl, 1995), to 15 times this bulk-earth ratio (i.e. , with the precision arbitrarily assumed to be 50 % of the bulk-earth-derived value). The resulting array of corrected ages and their respective uncertainties was converted to probability density functions (PDFs) assuming a Gaussian distribution and summed per patch in order to compare with isochron dating results. Note that this approach was primarily chosen to visualise a realistic range of corrected ages and is not equivalent to similar probabilistic methods using kernel density estimates (Weij et al., 2020).
Isochrons were constructed by applying least squares regressions in a three-dimensional space of (), () and () (Ludwig and Titterington, 1994). Isochron ages were calculated from the () and () intercept ± the 95 % confidence interval of the respective regression. The calculations were executed using a Python 3.9 implementation of the relevant regression models from IsoplotR (Vermeesch, 2018; Python code available upon request).
3.4 Morphological analysis
The cleaned samples were examined using a Keyence VHX-6000 digital microscope. To investigate the surface texture of CCCs, selected samples were gold coated and analysed using a JEOL JSM-6010LV scanning electron microscope. Secondary electron images were acquired using 15 kV accelerating voltage.
4.1 Sample characterisation
The grain size of CCCs in Water Icicle Close Cavern varies within each patch, but most specimens are approximately 1–10 mm in diameter. One out of the three sampled patches also contains larger crystals up to 30 mm in diameter. Each occurrence comprises a variety of crystal morphologies, as is typical for CCCs (Žák et al., 2018). Prevalent shapes include rhombohedra (Fig. 1a) and aggregates of crystals (Fig. 1b). Some specimens comprise elongated sheets or needles branching radially from a common centre point and show an almost spherulitic shape. Secondary electron microscopy (SEM) images reveal that rhombohedra-like morphologies have comparably smooth, stepped surfaces that mimic the overall rhombohedral geometry (Fig. 1c). More complex grains, on the other hand, have a highly irregular surface, but elongated rhombohedral shapes can still be recognised (Fig. 1d).
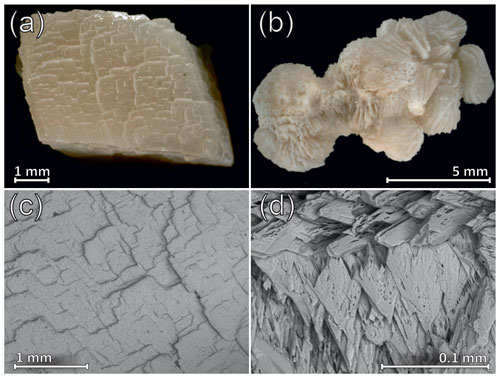
Figure 1Selected CCC morphologies of patch 1. (a) Rhombohedral calcite crystal with a stepped surface, (b) complex intergrown crystals composed of radially fibrous calcite, (c) SEM image of the surface of the sample depicted in panel (a) and (d) SEM image of panel (b).
The stable isotope composition of CCCs from patch 1 varies systematically with crystal morphology (Fig. 2). Rhombohedral crystals have δ18O values from −12.4 ‰ to −14.1 ‰ and δ13C values from −6.4 ‰ to −5.4 ‰. In comparison, complex grains show lower δ18O (−14.2 ‰ to −15.4 ‰) and higher δ13C (−5.1 ‰ to −4.5 ‰) values.
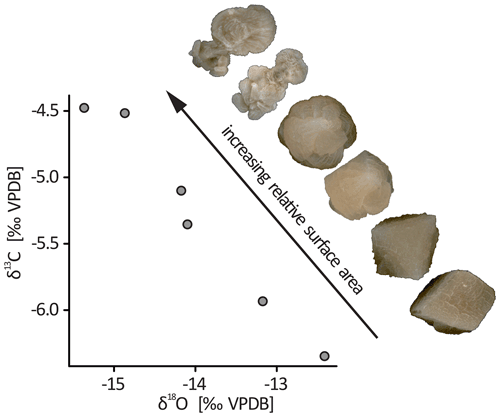
Figure 2Stable isotope compositions of selected crystals from patch 1. The data follow a trend with a negative slope, which is typical for CCCs. The stable isotope composition changes systematically with the progression from rhombohedral to radially branching morphologies. All depicted samples are between 2 and 5 mm in diameter, but the images were rescaled for better comparability.
These observations can be interpreted in the context of the genetic model of CCCs (Žák et al., 2004). As water freezes, the δ18O of residual water lowers progressively because 18O is preferentially incorporated into the growing ice. Conversely, δ13C increases due to the preferential loss of 12C as CO2 degasses. This trend is well established for CCCs (Žák et al., 2008, 2018) and is also observed from core to rim within single CCC grains (Töchterle, 2018).
The concept of a morphological evolution of CCCs has been formulated in previous studies (Žák et al., 2012), but analytical evidence supporting it has been lacking. The observed correlation between crystal morphology and stable isotope composition (Fig. 2) indicates that these CCCs formed during a single freezing event. Furthermore, complex aggregates apparently formed later in the freezing process than rhombohedral grains.
During freezing, it is not only the stable isotope composition that changes, but also the overall concentration of solutes. A large body of literature confirms the effects of thermodynamic variables such as supersaturation, ionic strength and the concentration of foreign ions or organic compounds on calcite morphologies and fabrics (e.g. Frisia et al., 2000; Sunagawa, 2005; Sand et al., 2012; Frisia, 2015; Hong et al., 2016; Mercedes-Martín et al., 2021). The morphological variety of CCCs observed in this study can be viewed as an expression of changing crystallisation driving forces in response to progressive freezing. Two fundamental pathways can alter the shape of a grain. Firstly, multiple individual crystals can intergrow to form crystal aggregates. Secondly, the crystal fabric can be influenced by various degrees of non-crystallographic branching in response to fast growth and/or high supersaturation of the parent solution, resulting in radial growth, with the endmember being a spherulite (Sunagawa, 2005; Shtukenberg et al., 2012; De Yoreo et al., 2015). Crucially for this study, by building a more complex geometry or creating a rougher surface, both of these mechanisms potentially increase the relative surface area of a grain (i.e. the ratio between surface area and volume).
4.2 230ThU analyses
Overall, 26 analyses were carried out on CCCs from three patches (Table 1). From each patch, three samples of three morphological types were selected. All analyses yielded high concentrations of 238U (1035±1 to 3843±5 ng g−1) and mostly low amounts of detrital Th (indicated by higher values of ). The values found in CCCs in Water Icicle Close Cavern are mostly comparable in order of magnitude to the values other studies have reported for CCCs (i.e. from approximately 10 to 10 000, e.g. Žák et al., 2009; Chaykovskiy et al., 2014; Spötl and Cheng, 2014; Munroe et al., 2021).
In the following sections, samples are visually grouped into three types (A, B and C) on the basis of crystal morphology and relative surface area. The results from each patch are discussed individually.
4.2.1 Patch 1
The samples selected from patch 1 comprise rhombohedral single crystals (morphology A), branched aggregates (morphology B), and also a set of transitional forms that show a progression from lower to higher degrees of non-crystallographic branching (morphology C, Fig. S1 in the Supplement). In this patch, varies by more than 1 order of magnitude (from 7.7±0.2 to 150±3) and correlates with the morphology type whereby the complex morphologies B and C show lower values (Table 1) indicative of higher 230Th0. More complex morphologies therefore yielded older uncorrected ages. The difference between these uncorrected ages is as large as ∼6200 years (Fig. 3).
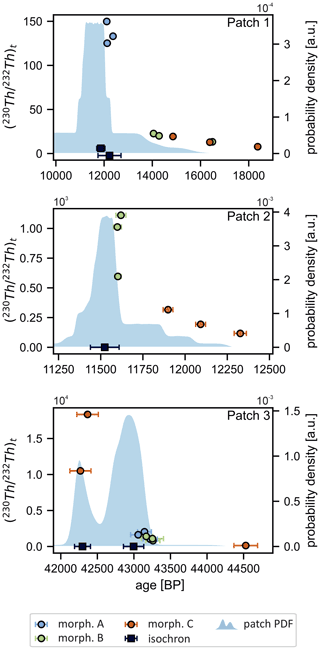
Figure 3Uncorrected ages plotted against the respective ratios. Colour represents morphology type. Also shown for each patch are corresponding isochron ages (±95 % confidence interval) and the summed normalised probability density functions (PDFs) calculated from an array of values. Samples of type A in patch 2, which are much older, are not shown to allow better scaling.
Given the substantial spread in nominal ages and lack of constraints on , it is tempting to infer distinct formation events for the different morphological types. However, CCCs in this patch conform to the morphological evolution described in Sect. 4.1, implying that they likely formed from the same parent solution during a single freezing event. It seems unlikely, however, that such an event lasted several thousand years and that the cave temperature remained stable throughout this entire period. The question arises of whether it is possible to find a ratio that would result in overlapping corrected ages, supporting the hypothesis that all samples formed at the same time and thus reconciling dating results with morphological observations.
We calculated an array of corrected ages based on multiple possible ratios (30 ratios between 1× and 15× of the bulk-earth-derived value) and derived a summed PDF (shaded area in Fig. 3) representing the cumulative distribution of all corrected ages in this patch. Naturally, samples with low are highly sensitive to and yield very wide distributions compared to samples with >100.
Figure 4 shows the 238U-normalised activities of 230Th and 232Th. These variables correlate with an R2 of 0.98 (r1.1 in Fig. 4, regression parameters in Table S1 in the Supplement), meaning that 98 % of the observed variance in () (which mainly determines the ages of these samples) can be explained by different levels of 232Th. From the least-squares regression, it is possible to calculate an isochron age (12 224±478 BP) and a activity ratio of 2.7±0.2. This result partly overlaps with the peak of the PDF. However, the linear regression model strongly depends on a single data point in the upper right hand corner of Fig. 4 (i.e. sample PT33). When disregarding this data point, the isochron age shifts to 11 865±172 BP (r1.2, R2=0.997) with a of 3.4±0.1, improving the agreement between the isochron and PDF ages. The isochron age of r1.2 also intercepts the high- samples (which are less sensitive to differences in the value) notably better. Excluding samples with very high levels of 230Th0 may be justified in cases where multiple sources of 230Th0 were mixed with varying ratios, as will be discussed in Sect. 4.3.
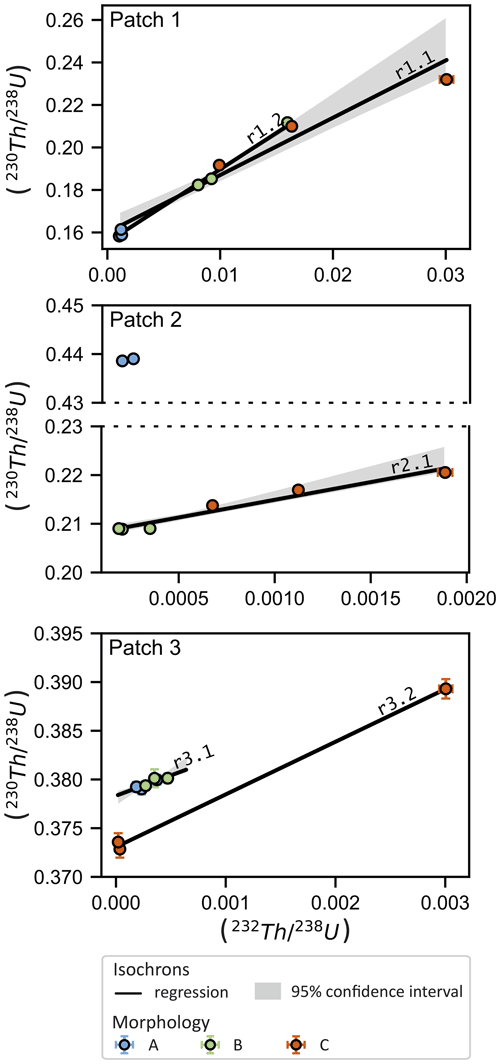
Figure 4Isochron plots of 238U-normalised thorium measurements of each CCC patch. Colour represents morphological type. The shaded area represents the 95 % confidence interval of the regression slope and intercept. Regression lines are labelled with an identification number corresponding to Table S1.
4.2.2 Patch 2
Patch 2 also contains morphologies of variable relative surface area (Fig. S2). Samples of morphology A are translucent with a brownish hue and tend to form smooth, euhedral crystal faces. Morphology B is milky white and shows a high degree of non-crystallographic branching close to spherulitic growth. Morphology C also has a milky-white colour but is characterised by complex aggregates of blocky crystals with a large relative surface area per grain.
Just as for patch 1, the uncorrected ages vary for each morphological type (Table 1). Types B and C cluster around 11 600 and 12 100 BP, respectively, while type A yielded much older ages of around 37 000 BP. Compared to patch 1, samples generally have higher ( values (117±2 to 2176±44), indicating relatively low 230Th0. Thus, correcting for 230Th0 yields a much narrower PDF sum (Fig. 3), and sub-sample ages for types A and B are identical within the uncertainty for each respective morphology. Again, a relationship between morphology and can be observed, where samples with a smaller relative surface area (morphologies A and B) are less affected by 230Th0 contamination than the most complex morphology (type C).
The dating results show that there are two distinct generations of CCC present in this patch (Table 1, Fig. 4). Types B and C plot along a common regression line (r2.1, R2=0.98), whereas type A samples are offset. For types B and C, the resulting isochron yields an age of 11 524±85 BP, indistinguishable from the PDF peak (Fig. 3), and a activity ratio of 7.3±0.7.
Note that the isochron-derived value for is approximately 9 times higher than the bulk-earth-derived value, meaning that the correction for 230Th0 is proportionally larger than that for patch 1, especially for samples with even moderately elevated . Previous U-series dating studies have proposed threshold values of >100 or even >300 (Li et al., 1989; Fensterer et al., 2010), above which detrital Th contamination is deemed to have an insignificant effect on the accuracy of dating results. Results from patch 2 indicate that in cases where the true value of is multiple times higher than the estimate of the bulk-earth-derived value, threshold values may need to be adjusted to even higher levels (potentially as high as >500 for relatively young samples) in order to achieve the same level of accuracy. However, we argue that such limits should be considered guides, and that each dataset should be evaluated on a case-by-case basis, as acceptable limits will be affected by age, the relationship between the analytical uncertainty and the uncertainty in 230Th0, and the age accuracy needed to address the question at hand. As presented here for CCCs, isochrons can be a useful and reliable approach if high-quality, reliably dateable samples with high are not available.
4.2.3 Patch 3
In patch 3, morphological types A and B consist of translucent calcite with a brownish hue (Fig. S3, similar to type A of patch 2). Type A resembles rhombohedral single crystals with subtle non-crystallographic branching, while type B branches more strongly, giving the grains a dumbbell-like shape. Type C is significantly larger (up to 30 mm in diameter) and shows a transition from the brownish rhombohedral type to milky-white calcite with a radially fibrous texture indicative of high degrees of non-crystallographic branching.
The U and Th concentrations of types A and B are similar to within an order of magnitude (Table 1), with high values (807±16 to 2042±41) indicating very clean samples with minimal 230Th0 contamination. The resulting uncorrected ages of these morphological types are identical within analytical uncertainty (Fig. 3).
In contrast, morphological type C shows an extreme range of values. The two large specimens, where aliquots from the cores of the samples were analysed (Fig. S3), have extremely high (10 505 and 18 343, respectively), yielding a very narrow PDF sum that is indistinguishable from the uncorrected ages. The third aliquot, however, which was a piece of surface material chipped off a larger type C grain, yielded the lowest (130±3) of this patch and hence an uncorrected age ∼2000 years older.
For this patch, the PDF sum clearly shows a bimodal distribution of corrected ages (Fig. 3). An isochron regression can be fitted to the data when exempting morphology C (r3.1, R2=0.89). The resulting isochron age of 43 065±139 BP ( , Fig. 4c) agrees with the older PDF peak corresponding to types A and B. The isochron age of type C (r3.2, 42 297±109 BP) also confirms the results of the drilled aliquots, and the corresponding (5.4±0.2) is similar to the value obtained from types A and B. However, it should be noted that the regression is largely controlled by a single highly inaccurate sample (PT24). Disregarding this sample, a very steep regression with (i.e. approx. 21× than the bulk-earth-derived value) would be necessary to connect the two clusters of data. Consequently, it is reasonable to propose two distinct generations of CCC at 43 065±139 BP (types A and B) and 42 297±109 BP (type C). The isochron approach is thus able to also resolve differing formation ages within a single patch.
4.3 Implications
The values derived from CCC isochrons are a complex function of different Th sources, including detrital particles, colloidal phases, organic compounds and dissolved carbonate species, which contribute to 230Th0 by adsorption or co-precipitation. All of the values we found (2.7±0.2 to 7.2±0.7, Table S1) are comparable to literature data on common speleothems (e.g. Beck et al., 2001; Hubert et al., 2006; Hoffmann et al., 2010; Carolin et al., 2013; Moseley et al., 2014; Arienzo et al., 2015; Carolin et al., 2016). However, there is significant variability in across the three patches. Other studies have reported a much lower in-cave variability with respect to in sediments, speleothems and drip water (Olley et al., 1997; Kaufman et al., 1998). A possible explanation for this high variability can be entertained by considering the dynamic nature of CCC-forming pools. Munroe et al. (2021) reported that the residual pools holding CCCs in Winter Wonderland Cave show different degrees of discolouration of the residual water, indicating that progressive freezing altered the physio-chemical properties of the residual water. One possible explanation could be that the ratio between the different Th sources listed above changes, thereby altering the Th isotopic composition. Non-linear behaviour of could also explain why the isochron approach in patch 1 agrees better with the PDF sum when sample PT33 is excluded from the regression. At this point, however, it remains unclear whether progressive freezing alters . Future investigations addressing this question may benefit from using trace element concentrations to distinguish between different sources of 230Th0 (e.g. Henderson and Slowey, 1998).
We have shown that for distinct patches of CCCs, an apparent spread in corrected ages can be an artefact arising from the underestimation of in samples with substantial 230Th0. The data indicate that the relative surface area of a CCC grain correlates with its 232Th content, likely because more detrital material adsorbs onto a larger surface. Thus, CCCs with smaller surface areas will tend to require smaller corrections for 230Th0 and yield more accurate ages. Further evidence supporting this is provided by the drilled aliquots of core material from patch 3, which show the highest values of all analyses and consequently yield ages that are not sensitive to correction.
The dating issues that we describe in this study are ultimately tied to sample preparation. Grain sizes of CCCs are highly variable and also seem to vary geographically. Very large specimens, sometimes over 10 cm in size, seem to be more prevalent in eastern European and Siberian caves (e.g. Chaykovskiy and Kadebskaya, 2015; Dublyansky, unpublished data), while studies from central Europe and the European Alps report mostly mm-sized crystals (Luetscher et al., 2013; Spötl and Cheng, 2014; Richter et al., 2014, 2015; Pavuza and Spötl, 2017; Richter et al., 2017; Colucci et al., 2017; Žák et al., 2018; Richter et al., 2019; Koltai et al., 2021; Richter et al., 2021; Spötl et al., 2021; Kluge et al., 2014). Drilling aliquots to obtain core material from small grains is unfeasible in practice, which consequently demands a critical assessment method. If high-quality samples with high are not available, dating multiple morphological types (if present) and constructing isochrons may be a key strategy for obtaining accurate and precise CCC chronologies.
-
The morphological variability of CCCs in Water Icicle Close Cavern arises from aggregation and varying degrees of non-crystallographic branching, which in turn reflect changes in the chemical environment during the CCC-forming freezing process. Samples range from rhombohedral single crystals that form early in the process to highly branched crystal aggregates that form when the driving forces of crystallisation increased at a later stage.
-
Patches of CCCs likely formed during a single freezing event if (a) the CCC morphologies show progressive non-crystallographic branching and (b) aliquots define a highly correlated isochron.
-
An apparent spread in uncorrected ages can arise from varying degrees of contamination by 230Th0. To guarantee high accuracy of samples with substantial 230Th0, we recommend that ages from a single patch are further analysed using an isochron approach from which both the age of formation and can be deduced. Furthermore, age offsets can be minimised by avoiding surface material where grain size permits.
-
To reliably determine the age (range) of a patch of CCCs, it is necessary to analyse multiple CCC individuals and – if present – multiple morphologies.
These conclusions have implications for the application of CCCs in palaeoclimatology. If confirmed by studies of CCCs from other caves, the accuracy of some previously published results (corrected with nominal values of ) should be viewed with caution. Of particular concern are the ages reported for those studies for which reported values of are low. As a consequence, the climatic interpretation of some studies that used CCCs to reconstruct past permafrost conditions may need to be re-assessed. This applies in particular to studies that analysed whole grains as opposed to aliquots of core material. In addition to increasing the number of aliquots per CCC patch, we recommend applying isochron dating to increase the robustness of CCC-based palaeoclimate interpretations.
All data necessary to reproduce the results presented herein are provided in the text and Supplement. The Python 3.9 code for isochron calculations is available upon request from the corresponding author.
The supplement related to this article is available online at: https://doi.org/10.5194/gchron-4-617-2022-supplement.
PT performed analyses and prepared the manuscript. SDS and GEM performed additional calculations and contributed to manuscript preparation. XL contributed with additional analyses. RLE, CS, YD and JG were instrumental in the study design, directing analyses and preparing the manuscript.
The contact author has declared that none of the authors has any competing interests.
Publisher's note: Copernicus Publications remains neutral with regard to jurisdictional claims in published maps and institutional affiliations.
The authors extend their gratitude to Alan Brentnall for cave access and guiding, Natural England for permitting fieldwork and sampling, and Peter Schroedl and Dylan Parmenter for assistance with analyses at the University of Minnesota. We also thank Andy Freem for discovering the CCCs in Water Icicle Close Cavern as well as Robbie Shone for supporting field work, photography and discovering additional CCC samples. Furthermore, we greatly appreciate the review comments provided by Karel Žák and David Richards, which improved the manuscript greatly.
This paper was edited by Norbert Frank and reviewed by Karel Žák and David Richards.
Arienzo, M. M., Swart, P. K., Pourmand, A., Broad, K., Clement, A. C., Murphy, L. N., Vonhof, H. B., and Kakuk, B.: Bahamian speleothem reveals temperature decrease associated with Heinrich stadials, Earth Planet. Sc. Lett., 430, 377–386, https://doi.org/10.1016/j.epsl.2015.08.035, 2015.
Beck, J. W., Richards, D. A., Lawrence, R., Edwards, R. L., Silverman, B. W., Smart, P. L., Donahue, D. J., Hererra-Osterheld, S., Burr, G. S., Calsoyas, L., Timothy, A. J., Jull, and Biddulph, D.: Extremely Large Variations of Atmospheric 14C Concentration During the Last Glacial Period, Science, 292, 2453–2458, https://doi.org/10.1126/science.1056649, 2001.
Carolin, S. A., Cobb, K. M., Adkins, J. F., Clark, B., Conroy, J. L., Lejau, S., Malang, J., and Tuen, A. A.: Varied Response of Western Pacific Hydrology to Climate Forcings over the Last Glacial Period, Science, 340, 1564–1566, https://doi.org/10.1126/science.1233797, 2013.
Carolin, S. A., Cobb, K. M., Lynch-Stieglitz, J., Moerman, J. W., Partin, J. W., Lejau, S., Malang, J., Clark, B., Tuen, A. A., and Adkins, J. F.: Northern Borneo stalagmite records reveal West Pacific hydroclimate across MIS 5 and 6, Earth Planet. Sc. Lett., 439, 182–193, https://doi.org/10.1016/j.epsl.2016.01.028, 2016.
Chaykovskiy, I. I. and Kadebskaya, O.: Morphology of cryogenic calcite from Rossiyskaya cave (Central Ural): Problems of mineralogy, petrography and metallogeny, Scientific readings in memory of P.N. Chirvinsky, 18, 102–112, 2015 (in Russian).
Chaykovskiy, I. I., Kadebskaya, O., and Žák, K.: Morphology, composition, age and origin of carbonate spherulites from caves of Western Urals, Geochem. Int., 52, 336–346, https://doi.org/10.1134/S0016702914020049, 2014.
Cheng, H., Adkins, J. F., Edwards, R. L., and Boyle, E. A.: U-Th dating of deep-sea corals, Geochim. Cosmochim. Ac., 64, 2401–2416, https://doi.org/10.1016/S0016-7037(99)00422-6, 2000.
Cheng, H., Edwards, R. L., Shen, C.-C., Polyak, V. J., Asmerom, Y., Woodhead, J., Hellstrom, J., Wang, Y., Kong, X., Spötl, C., Wang, X., and Calvin Alexander, E.: Improvements in 230Th dating, 230Th and 234U half-life values, and U–Th isotopic measurements by multi-collector inductively coupled plasma mass spectrometry, Earth Planet. Sc. Lett., 371–372, 82–91, https://doi.org/10.1016/j.epsl.2013.04.006, 2013.
Clark, C. D., Hughes, A. L.C., Greenwood, S. L., Jordan, C., and Sejrup, H. P.: Pattern and timing of retreat of the last British-Irish Ice Sheet, Quaternary Sci. Rev., 44, 112–146, https://doi.org/10.1016/j.quascirev.2010.07.019, 2012.
Cobb, K. M., Charles, C. D., Cheng, H., Kastner, M., and Edwards, R. L.: U/Th-dating living and young fossil corals from the central tropical Pacific, Earth Planet. Sc. Lett., 210, 91–103, https://doi.org/10.1016/S0012-821X(03)00138-9, 2003.
Colucci, R. R., Luetscher, M., Forte, E., Guglielmin, M., Lenaz, D., Princivalle, F., and Vita, F.: First alpine evidence of in Situ voarse cryogenic cave carbonates (CCC_coarse), Geogr. Fis. Din. Quat., 40, 53–59, 2017.
De Yoreo, J. J., Gilbert, P. U. P. A., Sommerdijk, N. A. J. M., Penn, R. L., Whitelam, S., Joester, D., Zhang, H., Rimer, J. D., Navrotsky, A., Banfield, J. F., Wallace, A. F., Michel, F. M., Meldrum, F. C., Cölfen, H., and Dove, P. M.: Crystallization by particle attachment in synthetic, biogenic, and geologic environments, Science, 349, aaa6760, https://doi.org/10.1126/science.aaa6760, 2015.
Dorale, J. A., Edwards, R. L., Alexander, E. C., Shen, C.-C., Richards, D. A., and Cheng, H.: Uranium-Series Dating of Speleothems: Current Techniques, Limits, & Applications, in: Studies of Cave Sediments: Physical and Chemical Records of Paleoclimate, edited by: Sasowsky, I. D. and Mylroie, J. E., Kluwe Academic/Plenum Publishers, 177–197, https://doi.org/10.1007/978-1-4419-9118-8_10, 2004.
Dublyansky, Y., Moseley, G. E., Lyakhnitsky, Y., Cheng, H., Edwards, R. L., Scholz, D., Koltai, G., and Spötl, C.: Late Palaeolithic cave art and permafrost in the Southern Ural, Scientific Reports, 8, 12080, https://doi.org/10.1038/s41598-018-30049-w, 2018.
Edwards, R. L., Chen, J. H., and Wasserburg, G. J.: 238U–234U–230Th–232Th systematics and the precise measurement of time over the past 500,000 years, Earth Planet. Sc. Lett., 81, 175–192, https://doi.org/10.1016/0012-821X(87)90154-3, 1987.
Fensterer, C., Scholz, D., Hoffmann, D., Mangini, A., and Pajón, J. M.: -dating of a late Holocene low uranium speleothem from Cuba, Geochim. Cosmochim. Ac., 9, 12015, https://doi.org/10.1088/1755-1315/9/1/012015, 2010.
Frisia, S.: Microstratigraphic logging of calcite fabrics in speleothems as tool for palaeoclimate studies, Int. J. Speleol., 44, 1–16, https://doi.org/10.5038/1827-806X.44.1.1, 2015.
Frisia, S., Borsato, A., Fairchild, I. J., and McDermott, F.: Calcite Fabrics, Growth Mechanisms, and Environments of Formation in Speleothems from the Italian Alps and Southwestern Ireland, J. Sediment. Res., 70, 1183–1196, https://doi.org/10.1306/022900701183, 2000.
Gascoyne, M.: Palaeoclimate determination from cave calcite deposits, Quaternary Sci. Rev., 11, 609–632, https://doi.org/10.1016/0277-3791(92)90074-I, 1992.
Gunn, J., Fairchild, I. J., Moseley, G. E., Töchterle, P., Ashley, K. E., Hellstrom, J., and Edwards, R. L.: Palaeoenvironments in the central White Peak District (Derbyshire, UK): evidence from Water Icicle Close Cavern, Cave and Karst Science, 47, 153–168, 2020.
Hellstrom, J.: U–Th dating of speleothems with high initial 230Th using stratigraphical constraint, Quat. Geochronol., 1, 289–295, https://doi.org/10.1016/j.quageo.2007.01.004, 2006.
Henderson, G. M. and Slowey, N. C.: U-Th Isochron Dating of the Marine Oxygen-Isotope Record, Mineral. Mag., 62A, 602–603, 1998.
Henderson, G. M. and Slowey, N. C.: Evidence from U–Th dating against Northern Hemisphere forcing of the penultimate deglaciation, Nature, 404, 61–66, https://doi.org/10.1038/35003541, 2000.
Hirose, K., Kikawada, Y., and Igarashi, Y.: Temporal variation and provenance of thorium deposition observed at Tsukuba, Japan, J. Environ. Radioactiv., 108, 24–28, https://doi.org/10.1016/j.jenvrad.2011.10.004, 2012.
Hoffmann, D. L., Beck, J. W., Richards, D. A., Smart, P. L., Singarayer, J. S., Ketchmark, T., and Hawkesworth, C. J.: Towards radiocarbon calibration beyond 28ka using speleothems from the Bahamas, Earth Planet. Sc. Lett., 289, 1–10, https://doi.org/10.1016/j.epsl.2009.10.004, 2010.
Hong, M., Xu, J., and Teng, H. H.: Evolution of calcite growth morphology in the presence of magnesium: Implications for the dolomite problem, Geochim. Cosmochim. Ac., 172, 55–64, https://doi.org/10.1016/j.gca.2015.09.022, 2016.
Hubert, A., Bourdon, B., Pili, E., and Meynadier, L.: Transport of radionuclides in an unconfined chalk aquifer inferred from U-series disequilibria, Geochim. Cosmochim. Ac., 70, 5437–5454, https://doi.org/10.1016/j.gca.2006.08.008, 2006.
Jaffey, A. H., Flynn, K. F., Glendenin, L. E., Bentley, W. C., and Essling, A. M.: Precision Measurement of Half-Lives and Specific Activities of 235U and 238U, Phys. Rev. C, 4, 1889–1906, https://doi.org/10.1103/PhysRevC.4.1889, 1971.
Kaufman, A., Wasserburg, G. J., Porcelli, D., Bar-Matthews, M., Ayalon, A., and Halicz, L.: U-Th isotope systematics from the Soreq cave, Israel and climatic correlations, Earth Planet. Sc. Lett., 156, 141–155, https://doi.org/10.1016/S0012-821X(98)00002-8, 1998.
Kluge, T., Affek, H. P., Zhang, Y. G., Dublyansky, Y., Spötl, C., Immenhauser, A., and Richter, D. K.: Clumped isotope thermometry of cryogenic cave carbonates, Geochim. Cosmochim. Ac., 126, 541–554, https://doi.org/10.1016/j.gca.2013.11.011, 2014.
Koltai, G., Spötl, C., Jarosch, A. H., and Cheng, H.: Cryogenic cave carbonates in the Dolomites (northern Italy): insights into Younger Dryas cooling and seasonal precipitation, Clim. Past, 17, 775–789, https://doi.org/10.5194/cp-17-775-2021, 2021.
Li, W.-X., Lundberg, J., Dickin, A. P., Ford, D. C., Schwarcz, H. P., McNutt, R., and Williams, D.: High-precision mass-spectrometric uranium-series dating of cave deposits and implications for palaeoclimate studies, Nature, 339, 534–536, https://doi.org/10.1038/339534a0, 1989.
Lin, J. C., Broecker, W. S., Hemming, S. R., Hajdas, I., Anderson, R. F., Smith, G. I., Kelley, M., and Bonani, G.: A Reassessment of U-Th and 14C Ages for Late-Glacial High-Frequency Hydrological Events at Searles Lake, California, Quaternary Res., 49, 11–23, https://doi.org/10.1006/qres.1997.1949, 1998.
Ludwig, K. R. and Titterington, D. M.: Calculation of isochrons, ages, and errors, Geochim. Cosmochim. Ac., 58, 5031–5042, https://doi.org/10.1016/0016-7037(94)90229-1, 1994.
Luetscher, M., Borreguero, M., Moseley, G. E., Spötl, C., and Edwards, R. L.: Alpine permafrost thawing during the Medieval Warm Period identified from cryogenic cave carbonates, The Cryosphere, 7, 1073–1081, https://doi.org/10.5194/tc-7-1073-2013, 2013.
Mercedes-Martín, R., Rogerson, M., Prior, T. J., Brasier, A. T., Reijmer, J. J. G., Billing, I., Matthews, A., Love, T., Lepley, S., and Pedley, M.: Towards a morphology diagram for terrestrial carbonates: Evaluating the impact of carbonate supersaturation and alginic acid in calcite precipitate morphology, Geochim. Cosmochim. Ac., 306, 340–361, https://doi.org/10.1016/j.gca.2021.04.010, 2021.
Moore, W. S.: The thorium isotope content of ocean water, Earth Planet. Sc. Lett., 53, 419–426, https://doi.org/10.1016/0012-821X(81)90046-7, 1981.
Moore, W. S. and Sackett, W. M.: Uranium and thorium series inequilibrium in sea water, J. Geophys. Res., 69, 5401–5405, https://doi.org/10.1029/JZ069i024p05401, 1964.
Moseley, G. E., Spötl, C., Svensson, A., Cheng, H., Brandstätter, S., and Edwards, R. L.: Multi-speleothem record reveals tightly coupled climate between central Europe and Greenland during Marine Isotope Stage 3, Geology, 42, 1043–1046, https://doi.org/10.1130/G36063.1, 2014.
Moseley, G. E., Spötl, C., Cheng, H., Boch, R., Min, A., and Edwards, R. L.: Termination-II interstadial/stadial climate change recorded in two stalagmites from the north European Alps, Quaternary Sci. Rev., 127, 229–239, https://doi.org/10.1016/j.quascirev.2015.07.012, 2015.
Munroe, J., Kimble, K., Spötl, C., Marks, G. S., McGee, D., and Herron, D.: Cryogenic cave carbonate and implications for thawing permafrost at Winter Wonderland Cave, Utah, USA, Scientific Reports, 11, 6430, https://doi.org/10.1038/s41598-021-85658-9, 2021.
Olley, J. M., Roberts, R. G., and Murray, A. S.: Disequilibria in the uranium decay series in sedimentary deposits at Allen's cave, nullarbor plain, Australia: Implications for dose rate determinations, Radiat. Meas., 27, 433–443, https://doi.org/10.1016/S1350-4487(96)00114-X, 1997.
Orvošová, M., Deininger, M., and Milovský, R.: Permafrost occurrence during the Last Permafrost Maximum in the Western Carpathian Mountains of Slovakia as inferred from cryogenic cave carbonate, Boreas, 43, 750–758, https://doi.org/10.1111/bor.12042, 2014.
Pavuza, R. and Spötl, C.: Neue Daten zu Vorkommen und Entstehung kryogener Calcite in ostalpinen Höhlen, Die Höhle, 68, 100–106, 2017.
Richards, D. A. and Dorale, J. A.: Uranium-series Chronology and Environmental Applications of Speleothems, Rev. Mineral. Geochem., 52, 407–460, https://doi.org/10.2113/0520407, 2003.
Richter, D. K. and Riechelmann, D. F.: Late Pleistocene cryogenic calcite spherolites from the Malachitdom Cave (NE Rhenish Slate Mountains, Germany): origin, unusual internal structure and stable C-O isotope composition, Int. J. Speleol., 37, 119–129, 2008.
Richter, D. K., Meissner, P., Immenhauser, A., Schulte, U., and Dorsten, I.: Cryogenic and non-cryogenic pool calcites indicating permafrost and non-permafrost periods: a case study from the Herbstlabyrinth-Advent Cave system (Germany), The Cryosphere, 4, 501–509, https://doi.org/10.5194/tc-4-501-2010, 2010.
Richter, D. K., Harder, M., Niedermayr, A., and Scholz, D.: Zopfsinter in der Zoolithenhöhle: Erstfund kryogener Calcite in der Fränkischen Alb (in German), Mitteilungen des Verbandes der deutschen Höhlen- und Karstforscher e.V., 60, 36–41, 2014.
Richter, D. K., Goll, K., Grebe, W., Niedermayr, A., Platte, A., and Scholz, D.: Weichselzeitliche Kryocalcite als Hinweise für Eisseen in der Hüttenbläserschachthöhle (Iserlohn/NRW), E&G Quaternary Sci. J., 64, 67–81, https://doi.org/10.3285/eg.64.2.02, 2015.
Richter, D. K., Knolle, F., Meyer, S., and Scholz, D.: Erste weichselzeitliche Kryocalcit-Vorkommen in Höhlen des Iberg/Winterberg Riffkomplexes (Harz), Mitteilungen des Verbandes der deutschen Höhlen- und Karstforscher e.V., 63, 52–57, 2017 (in German).
Richter, D. K., Scholz, D., Jöns, N., Neuser, R. D., and Breitenbach, S. F. M.: Coarse-grained cryogenic aragonite as end-member of mineral formation in dolomite caves, Sediment. Geol., 376, 136–146, https://doi.org/10.1016/j.sedgeo.2018.08.006, 2018.
Richter, D. K., Mueller, M., Platte, A., and Scholz, D.: Erste weichselzeitliche Kryocalcite im Attendorn-Elsper Riffkomplex (Frettermühler Wasserhöhle, Südwestfalen) (in German), Geologie und Paläontologie in Westfalen, 93, 1–16, 2019.
Richter, D. K., Schudelski, A., Neuser, R. D., and Scholz, D.: Weichelzeitliche Umbrellacalcite aus der Höhle “Malachitdom” (NE-Sauerland): vom Kaltwasser- zum Ausfrierstdium in Pools auf Eis, Geologie und Paläontologie in Westfalen, 94, 1–14, 2021 (in German).
Sand, K. K., Rodriguez-Blanco, J. D., Makovicky, E., Benning, L. G., and Stipp, S. L. S.: Crystallization of CaCO3 in Water–Alcohol Mixtures: Spherulitic Growth, Polymorph Stabilization, and Morphology Change, Cryst. Growth Des., 12, 842–853, https://doi.org/10.1021/cg2012342, 2012.
Scott, M. R.: Thorium and uranium concentrations and isotope ratios in river sediments, Earth Planet. Sc. Lett., 4, 245–252, https://doi.org/10.1016/0012-821X(68)90042-3, 1968.
Shen, C.-C., Wu, C.-C., Cheng, H., Edwards, R. L., Hsieh, Y.-T., Gallet, S., Chang, C.-C., Li, T.-Y., Lam, D. D., Kano, A., Hori, M., and Spötl, C.: High-precision and high-resolution carbonate 230Th dating by MC-ICP-MS with SEM protocols, Geochim. Cosmochim. Ac., 99, 71–86, https://doi.org/10.1016/j.gca.2012.09.018, 2012.
Shtukenberg, A. G., Punin, Y. O., Gunn, E., and Kahr, B.: Spherulites, Chem. Rev., 112, 1805–1838, https://doi.org/10.1021/cr200297f, 2012.
Skřivánek, F. (Ed.): Jeskyně na Chlumu v Českém krasu (Caves at Chlum in the Bohemian Karst), Československý kras, 7, 24–34, 1954 (in Czech).
Spötl, C.: Long-term performance of the Gasbench isotope ratio mass spectrometry system for the stable isotope analysis of carbonate microsamples, Rapid Commun. Mass Sp., 25, 1683–1685, https://doi.org/10.1002/rcm.5037, 2011.
Spötl, C. and Cheng, H.: Holocene climate change, permafrost and cryogenic carbonate formation: insights from a recently deglaciated, high-elevation cave in the Austrian Alps, Clim. Past, 10, 1349–1362, https://doi.org/10.5194/cp-10-1349-2014, 2014.
Spötl, C. and Vennemann, T. W.: Continuous-flow isotope ratio mass spectrometric analysis of carbonate minerals, Rapid Commun. Mass Sp., 17, 1004–1006, https://doi.org/10.1002/rcm.1010, 2003.
Spötl, C., Koltai, G., Jarosch, A. H., and Cheng, H.: Increased autumn and winter precipitation during the Last Glacial Maximum in the European Alps, Nat. Commun., 12, 1839, https://doi.org/10.1038/s41467-021-22090-7, 2021.
Sunagawa, I.: Crystals: Growth, morphology, and perfection, Cambridge University Press, Cambridge, United Kingdom, https://doi.org/10.1017/CBO9780511610349, 2005.
Töchterle, P.: Cryogenic cave carbonates from the Ural Mountains (Russia), MSc thesis, University of Innsbruck, Austria, https://doi.org/10.5281/zenodo.5807542, 2018.
UK METoffice: Climatic Research Unit (CRU) Time-Series (TS) version 4.04 of high-resolution gridded data of month-by-month variation in climate (Jan. 1901-Dec. 2019), Centre for Environmental Data Analysis [data set], https://catalogue.ceda.ac.uk/uuid/89e1e34ec3554dc98594a5732622bce9, last access: 6 October 2020.
Vermeesch, P.: IsoplotR: A free and open toolbox for geochronology, Chem. Geol., 9, 1479–1493, https://doi.org/10.1016/j.gsf.2018.04.001, 2018.
Wedepohl, H.: The composition of the continental crust, Geochim. Cosmochim. Ac., 59, 1217–1232, https://doi.org/10.1016/0016-7037(95)00038-2, 1995.
Weij, R., Woodhead, J., Hellstrom, J., and Sniderman, K.: An exploration of the utility of speleothem age distributions for palaeoclimate assessment, Quat. Geochronol., 60, 101112, https://doi.org/10.1016/j.quageo.2020.101112, 2020.
Žák, K., Onac, B. P., and Perşoiu, A.: Cryogenic carbonates in cave environments: A review, Quatern. Int., 187, 84–96, https://doi.org/10.1016/j.quaint.2007.02.022, 2008.
Žák, K., Hercman, H., Orvošová, M., and Jačková, I.: Cryogenic cave carbonates from the Cold Wind Cave, Nízke Tatry Mountains, Slovakia: Extending the age range of cryogenic cave carbonate formation to the Saalian, Int. J. Speleol., 38, 139–152, https://doi.org/10.5038/1827-806X.38.2.5, 2009.
Žák, K., Richter, D. K., Filippi, M., Živor, R., Deininger, M., Mangini, A., and Scholz, D.: Coarsely crystalline cryogenic cave carbonate – a new archive to estimate the Last Glacial minimum permafrost depth in Central Europe, Clim. Past, 8, 1821–1837, https://doi.org/10.5194/cp-8-1821-2012, 2012.
Žák, K., Urban, J., Cı́lek, V., and Hercman, H.: Cryogenic cave calcite from several Central European caves: Age, carbon and oxygen isotopes and a genetic model, Chem. Geol., 206, 119–136, https://doi.org/10.1016/j.chemgeo.2004.01.012, 2004.
Žák, K., Onac, B. P., Kadebskaya, O., Filippi, M., Dublyansky, Y., and Luetscher, M.: Cryogenic Mineral Formation in Caves, in: Ice caves, edited by: Perşoiu, A. and Lauritzen, S.-E., Elsevier, Amsterdam, Oxford, Cambridge, Mass., https://doi.org/10.1016/B978-0-12-811739-2.00035-8, 2018.